Arc Welding
This is a summary of a paper of the same title, authored by K. Májlinger, E. Kalácska, and P. Russo Spena, used by permission.M-65
Researchers at the Budapest University of Technology and Economics and the Free University of Bozen-Bolzano tested gas metal arc welding (GMAW) of dissimilar Advanced High-Strength Steel (AHSS) sheets.M-65 The test pieces were 100 x 50 mm samples of 1.4 mm TWIP (TWIP1000) and 0.9 mm TRIP (HCTC800T) sheet steels were welded in a lap joint configuration with 0.8 mm diameter AWS ER307Si austenitic stainless steel wire to determine appropriate GMAW parameters for good quality welds. Quality was determined by external appearance, microstructure, and mechanical properties. Good welds were achieved with linear heat inputs (Q) with ranges from 500-650 kJ/m. The only fractures that occurred appeared within the weld bead by ductile failure modes. The HAZ of the TWIP steel showed grain coarsening and the HAZ of the TRIP steel experienced microstructural changes relative to the distance from the fusion boundary. The ultimate tensile strength (UTS) varies between 73%-84% of the weaker of the two steels.
Welding was conducted with an automated linear drive system with pure Argon (99.996% Ar) shielding gas at 10L/min. Wire feed rates were approximately 3.5 m/min with Direct Current Electrode Positive (DCEP) polarity. Changes in current, voltage, weld speed, and the resulting linear energy are compared in Table 1.
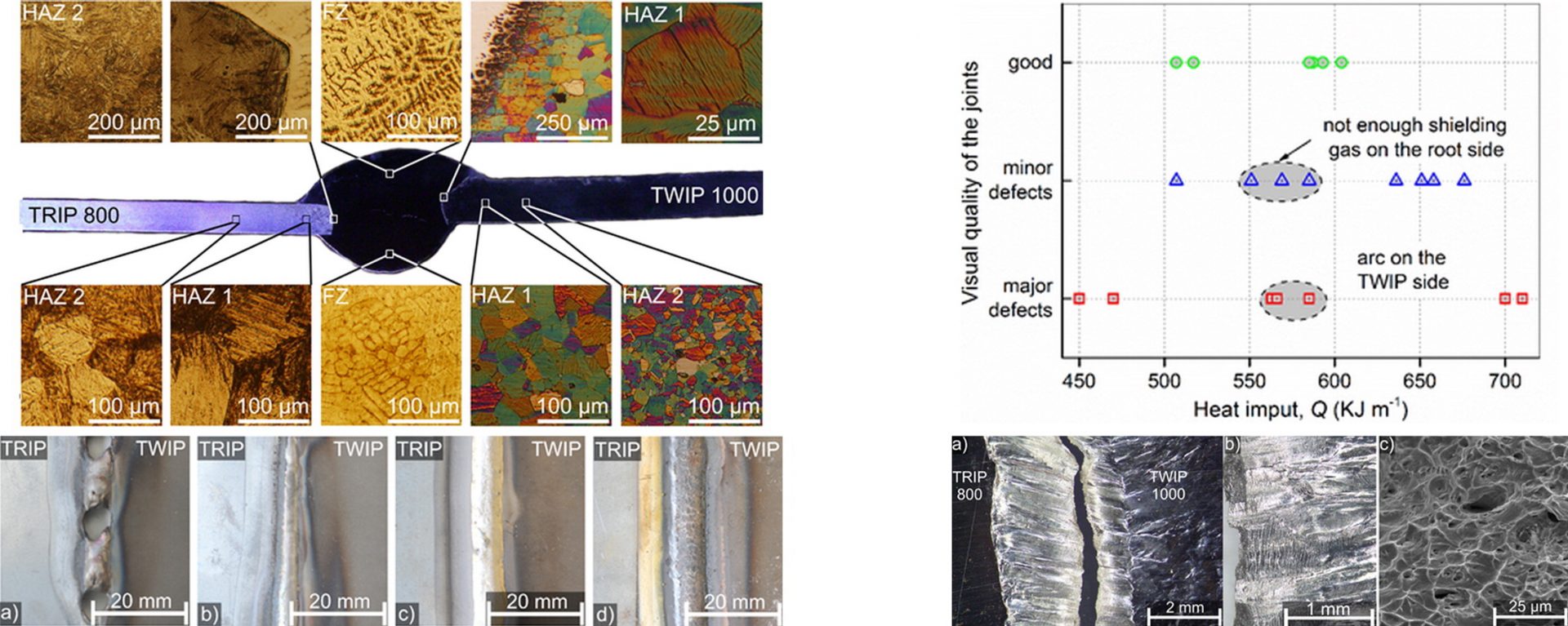
Figure 1: Overview of Dissimilar AHSS GMAW Welding.M-65
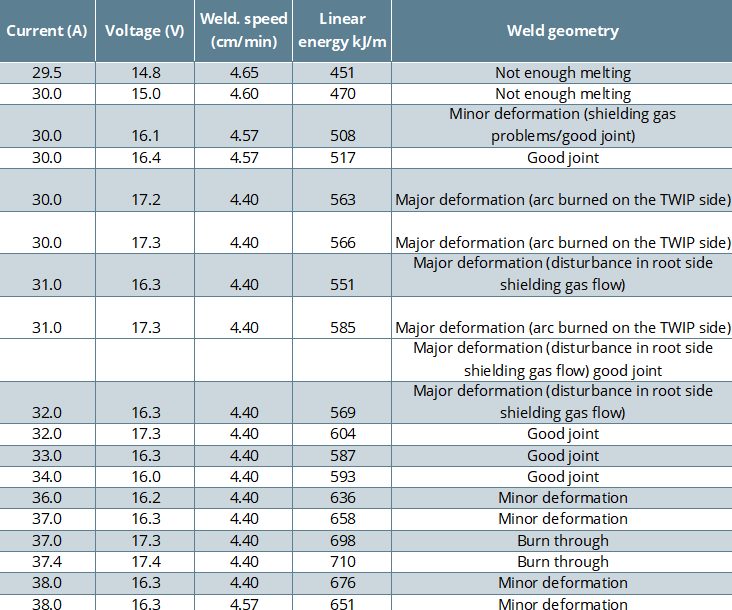
Table 1: Results of the preliminary welding tests in terms of TWIP-TRIP joint quality.M-65
After welding, transverse sections were cut from the welds and etched to show the microstructure. Vickers hardness testing was conducted on the weld samples based on the ASTM E384 standard. Tensile tests were performed on the samples according to the EN ISO 6892-1 standard. Tests were also conducted on unwelded TWIP and TRIP steels for comparison. Scanning electron microscopy (SEM) examinations were made of fracture surfaces to determine failure modes and examine for microscopic weld defects.
The study concluded that dissimilar welds between AHSS steels with the GMAW process can be achieved with consistent results desired for automotive applications.
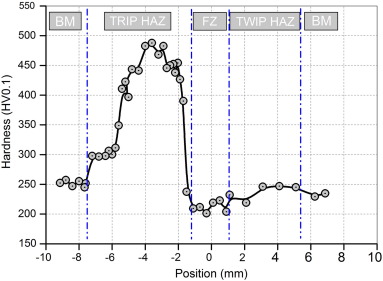
Figure 2: Vickers Hardness Across Weldment.M-65
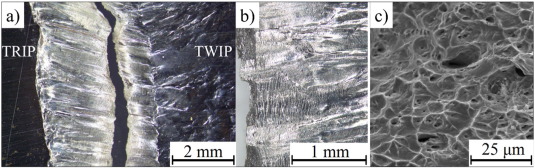
Figure 3: Ductile Failure in the fragile zones (FZ).M-65
2ndGen AHSS, AHSS, Steel Grades
TWinning Induced Plasticity (TWIP) steels have the highest strength-ductility combination of any steel used in automotive applications, with tensile strength typically exceeding 1000 MPa and elongation typically greater than 50%.
TWIP steels are alloyed with 12% to 30% manganese that causes the steel to be fully austenitic even at room temperature. Other common alloying additions include up to 3% silicon, up to 3% aluminum, and up to 1% carbon. Secondary alloying additions include chromium, copper, nitrogen, niobium, titanium, and/or vanadium.D-29 The high alloying levels and substantially greater levels of strength and ductility place these into the 2nd Generation of Advanced High Strength Steels. Furthermore, due to the density of the major alloying additions relative to iron, TWIP steels have a density which is about 5% lower than most other steels.
Calling this type of steel TWIP originates from the characteristic deformation mode known as twinning. Deformation twins produced during sheet forming leads to microstructural refinement and high values of the instantaneous hardening rate (n-value). The resultant twin boundaries act like grain boundaries and strengthen the steel. On either side of a twin boundary, atoms are located in mirror image positions as indicated in the schematic microstructure shown in Figure 1. Figure 2 highlights the microstructure of TWIP steel after annealing and after deformation.

Figure 1: Schematic of TWIP steel microstructure.

Figure 2: TWIP steel in the annealed condition (left) and after deformation (right) showing deformation twins. The number of deformation twins increases with increasing strain.K-42
EDDS or Interstitial-Free or Ultra-Low Carbon steels are different descriptions for the most formable lower-strength steel. Possible test results for this grade are 150 MPa yield strength, 300 MPa tensile strength, 22% to 25% uniform elongation, and 45% to 50% total elongation. In contrast, test results on TWIP steels may show 500 MPa yield strength, 1000 MPa tensile strength, 55% uniform elongation, and 60% total elongation.
The stress-strain curves for these two grades are compared in Figure 3. The TWIP curves show the manifestation of Dynamic Strain Aging (DSA), also known as the PLC effect, with more details to follow.

Figure 3: Uniaxial tensile stress-strain curves for an interstitial-free (IF) extra-deep-drawing steel and an austenitic Fe-18%Mn-0.6%C-1.5%Al TWIP steel. Curves are presented both terms of engineering (s,e) and true (σ,ε) stresses and strains, respectively.D-30
Figure 4 compares the results of bulge testing ferritic interstitial-free (IF) steel and austenitic Fe-18%Mn-0.6%C-1.5%Al TWIP steel. The TWIP steel is still undamaged at a dome height that is 31% larger than the IF steel dome height at failure.D-30

Figure 4: Comparison of dome testing between EDDS and TWIP.D-30
Excellent stretch formability is associated with high n-values. Shown in Figure 5 is a plot showing how the instantaneous n-value changes with applied strain. N-value increases to a value of 0.45 at an approximate true (logarithmic) strain of 0.2 and then remains relatively constant until an approximate true strain of 0.3 before increasing again. The high and uniform n-value delays necking and minimizes strain peaks. Twins continue to form at higher strains, leading to finer microstructural features and continued increases in n-value at higher strains.

Figure 5: Instantaneous n-value changes with applied strain. TWIP steels have high and uniform n-value leading to excellent stretch formability.C-30
A microstructural deformation phenomenon known as the Portevin-LeChatelier (PLC) effect occurs when deforming some TWIP steels to higher strain levels. The PLC effect is known by several other names as well, including jerky flow, discontinuous yielding, and dynamic strain aging (DSA).
The severity varies with alloy, strain rate, and deformation temperature. Figure 6 shows how DSA affects the appearance of the stress strain curve of two TWIP alloys.D-29 The primary difference in the alloy design is the curves on the right are for steel containing 1.5% aluminum, with the curves on the left for a steel without aluminum. The addition of aluminum delays the serrated flow until higher levels of strain. Note that both alloys have negative strain rate sensitivity.

Figure 6: Influence of aluminum additions on serrated flow in Fe-18%Mn-0.6%C TWIP (Al-free on the left) and Fe-18%Mn-0.6%C-1.5% Al TWIP (Al-added on the right).D-29
The primary macroscopic manifestations of the Portevin-LeChatelier (PLC) effect areD-29:
- negative strain rate sensitivity.
- stress-strain curve showing serrated or jerky flow, indicating non-uniform deformation. Strain localization takes place in propagating or static deformation bands.
- the strain rate within a localized band is typically one order of magnitude larger, while that outside the band is one order of magnitude lower, than the applied strain rate.
- limited post-uniform elongation, meaning uniform elongation is just below total elongation. Said another way, fracture occurs soon after necking initiation.
The PLC effect leads to relatively poor sheared edge expansion, as measured in a hole expansion test. Figure 7 on the left highlights the crack initiation site in a sample of highly formable EDDS-IF steel, showing the classic necking appearance with extensive thinning prior to fracture. In contrast, note the absence of necking in the TWIP steel shown in the right image in Figure 7.D-29
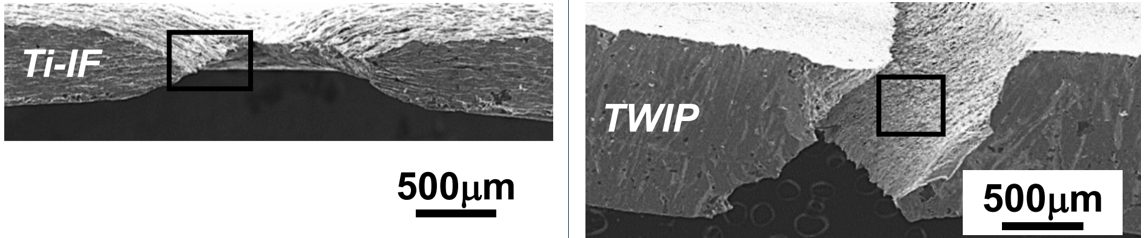
Figure 7: Sheared edge ductility comparison between IF (left) and TWIP (right) steel. TWIP steels lack the sheared edge expansion capability of IF steels.D-29
The stress-strain curves of several TWIP grades are compared in Figure 8.

Figure 8: Engineering stress-strain curve for several TWIP Grades.P-18
Complex-shaped parts requiring energy absorption capability are among the candidates for TWIP steel application, Figure 9.

Figure 9: Potential TWIP Steel Applications.N-24
Early automotive applications included the bumper beam of the 2011 Fiat Nuova Panda (Figure 10), resulting in a 28% weight savings and 22% cost savingsN-24 over the prior model which used a combination of PHS and DP steels.D-31

Figure 10: Transitioning to a TWIP Bumper Beam Resulted in Weight and Cost Savings in the 2011 Fiat Nuova Panda. N-24, D-31
In the 2014 Jeep Renegade BU/520, a welded blank combination of 1.3 mm and 1.8 mm TWIP 450/950 (Figure 11) replaced a two-piece aluminum component, aiding front end stability while reducing weight in a vehicle marketed for off-road applications.D-31

Figure 11: A TWIP welded blank improved performance and lowered weight in the 2014 Jeep Renegade BU/520.D-31
Also in 2014, the Renault EOLAB concept car where the A-Pillar Lower and the Sill Side Outer were stamped from TWIP 980 steel.R-21 By 2014, GM Daewoo used TWIP grades for A-Pillar Lowers and Front Side Members, and Hyundai used TWIP steel in 16 underbody parts. Ssangyong and Renault Samsung Motors used TWIP for Rear Side Members.I-20
Other applications include shock absorber housings, floor cross-members, wheel disks and rims, wheelhouses, and door impact beams.
A consortium called TWIP4EU with members from steel producers, steel users, research centers, and simulation companies had the goal of developing a simulation framework to accurately model the complex deformation and forming behavior of TWIP steels. The targeted part prototype component was a backrest side member of a front seat, Figure 12. Results were published in 2015.H-58

Figure 12: TWIP4EU Prototype Component formed from TWIP Steel.H-58
In addition to a complex thermomechanical mill processing requirements and high alloying costs, producing TWIP grades is more complex than conventional grades. Contributing to the challenges of TWIP production is that steelmaking practices need to be adjusted to account for the types and amounts of alloying. For example, the typical ferromanganese grade used in the production of other grades has phosphorus levels detrimental to TWIP properties. In addition, high levels of manganese and aluminum may lead to forming MnO and Al2O3 oxides on the surface after annealing, which could influence zinc coating adhesion in a hot dip galvanizing line.D-29