1stGen AHSS, 2ndGen AHSS, 3rdGen AHSS, AHSS, Blog, homepage-featured-top, main-blog, News, Production Managers, Steel Grades, Tool & Die Professionals
This month’s blog was contributed by Peter Ulintz, Precision Metalforming Association. This content originally appeared in the September 2023 issue of MetalForming Magazine under the title “Stronger AHSS Knowledge Required for Metal Stampers” and has been reproduced with the permission of MetalForming Magazine.
Metal stampers and die shops experienced with mild and HSLA steels often have problems making parts from AHSS grades. The higher initial yield strengths and increased work hardening of these steels can require as much as four times the working loads of mild steel. Some AHSS grades also have hardness levels approaching the dies used to form them.
Dies Get Tougher
Metal stampers and die shops experienced with mild and HSLA steels often have problems making parts from AHSS grades. The higher initial yield strengths and increased work hardening of these steels can require as much as four times the working loads of mild steel. Some AHSS grades also have hardness levels approaching the dies used to form them.
The higher stresses required to penetrate higher-strength materials require increased punch-to-die clearances compared to mild steels and HSLA grades. Why? This clearance acts as leverage to bend and break the slug out of the sheet metal. Stronger materials need longer levers to bend the slug. The required clearance is a function of the steel grade and tensile strength, and sheet thickness.
Increasing cutting clearance can result in punch cracking and head breakage due to higher snapthrough loads and reverse-unloading forces within the die. Adding shear angles to the punch face helps reduce punch forces and reverse unloading.
Tight-cutting clearances increase the tendency for die galling and chipping. The severity of galling depends on the surface finish and microstructure of both the tool steel and work material. Chipping can occur when process stresses are high enough to cause low-cycle fatigue of the tooling material, indicating that the material lacks toughness.
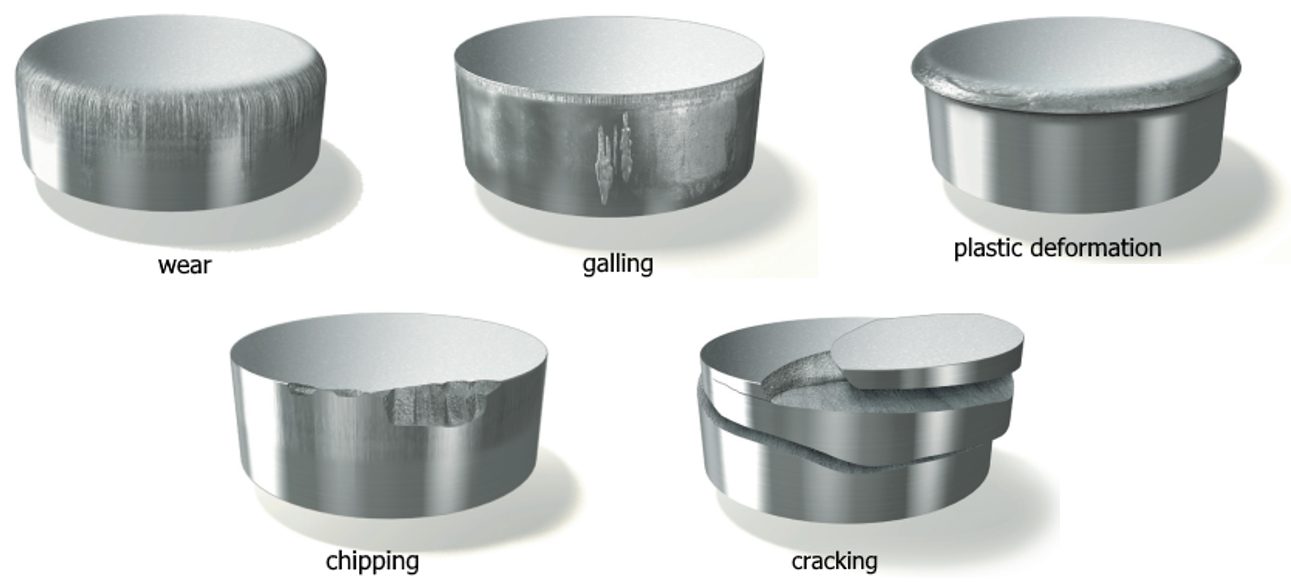
Stamping Tool Failure Modes (Citations T-20 and U-7)
Tempering of tools and dies represents a critical heat-treatment step and serves more than one purpose, but of primary concern is the need to relieve residual stresses and impart toughness. Dies placed in service without proper tempering likely will experience early failure.
Dies made from the higher-alloy tool-steel grades (D, M or T grades) require more than one tempering step. These grades contain large amounts of retained austenite and untempered martensite after the first tempering step and require at least one more temper to relieve internal stresses, and sometimes a third temper for even greater toughness.
Unfortunately, heat treatment remains a “black-box” process for most die shops and manufacturing companies, which send soft die details to the local heat treat facility, with hardened details returned. A cursory Rockwell hardness test may be conducted at the die shop when the parts return. If they meet hardness requirements, the parts usually are accepted, regardless of how they may have been processed—a problem, as hardness alone does not adequately measure impact toughness.
Machines Get Stronger
The increased forces needed to form, cut and trim higher-strength steels create significant challenges for pressroom equipment and tooling. These include excessive tooling deflections, damaging tipping-moments, and amplified vibrations and snapthrough forces that can shock and break dies—and sometimes presses. Stamping AHSS materials can affect the size, strength, power and overall configuration of every major piece of the press line, including material-handling equipment, coil straighteners, feed systems and presses.
Here is what every stamper should know about higher-strength materials:
- Because higher-strength steels require more stress to deform, additional servo motor power and torque capability may be needed to pull the coil material through the straightener. Additional back tension between the coil feed and straightening equipment also may be required due to the higher yield strength of the material in the loop as the material tries to push back against the straightener and feed system.
- Higher-strength materials, due to their greater yield strengths, have a greater tendency to retain coil set. This requires greater horsepower to straighten the material to an acceptable level of flatness. Straightening higher-strength coils requires larger-diameter rolls and wider roll spacing in order to work the stronger material more effectively. But increasing roll diameter and center distances on straighteners to accommodate higher-strength steels limits the range of materials that can effectively be straightened. A straightener capable of processing 600-mm-wide coils to 10 mm thick in mild steel may still straighten 1.5-mm-thick material successfully. But a straightener sized to run the same width and thickness of DP steel might only be capable of straightening 2.5 mm or 3.0-mm thick mild steel. This limitation is primarily due to the larger rolls and broadly spaced centers necessary to run AHSS materials. The larger rolls, journals and broader center distances safeguard the straightener from potential damage caused by the higher stresses.
- Because higher-strength materials require greater stress to blank and punch as compared to HSLA or mild steel, they generate proportionally increased snapthrough and reverse-unloading forces. High-tensile snapthrough forces introduce large downward accelerations to the upper die half. These forces work to separate the upper die from the bottom of the ram on every stroke. Insufficient die-clamping force could cause the upper-die half to separate from the bottom of the ram on each stroke, causing fatigue to the upper-die mounting fasteners.
- Because energy is expended with each stroke of the press—and this energy must be replaced—critical attention must focus on the size (horsepower) of the main drive motor and the rotational speed of the flywheel in higher-strength-steel applications. The main motor, with its electrical connection, provides the only source of energy for the press and it must generate sufficient power to meet the demands of the stamping operation. The motor must be properly sized to replace the increased energy expended during each press stroke. For these reasons, some stampers consider the benefits of servo-driven presses for these applications.
As steels becomes stronger, a corresponding increase in process knowledge is required in terms of die design, construction and maintenance, and equipment selection.
You can read more about these topics at these links:
Tooling and Die Wear
Coil Processing Straightening and Leveling
Press Requirements
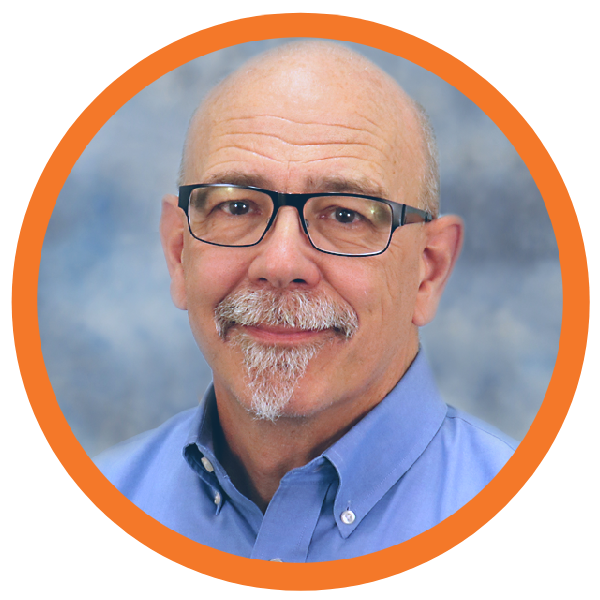
Thanks go to Peter Ulintz, of the Precision Metalforming Association (PMA) for authoring this article. Ulintz was employed in the metal stamping and tool & die industries for 38 years before joining Precision Metalforming Association (PMA) in 2015. He provides industry-related training and seminars in Stamping Press Operation and Setup; Designing and Building Metal Stamping Dies; Die Maintenance and Troubleshooting; Metal Stamping Design for Manufacturability; Deep Draw Tooling and Process Technology; Stamping Higher Strength Steels; and Problem Solving in the Press Shop. Peter is a contributor to ASM Handbook, Volume 14B, Metalworking: Sheet Forming (2006) and writes the monthly column, Tooling by Design, for PMA’s monthly publication, MetalForming Magazine.
News
To understand the difference between localized and global fractures, you must first understand strain gradients (see the article in our blog, AHSS Strain Hardening and Gradients). Gradients can result in highly concentrated strains (peak strain condition) that typically occurs in an embossment or character line where the deformation mode is in plane strain. Peak strains can develop rapidly in a very localized area (Figure 1). Under additional loads, this can result in the onset of localized necking, which means the material has reached its tensile strength and will fail at its weakest point or highest strain. When a slight increase in strain is applied, the material will fracture, sometimes at deformation levels less than predicted. This condition can be found in AHSS products, where multiple phases exist within the steel’s microstructure, each with different properties. A global fracture also typically occurs in plane strain, but more commonly down a sidewall or other area with more moderate geometry complexity.
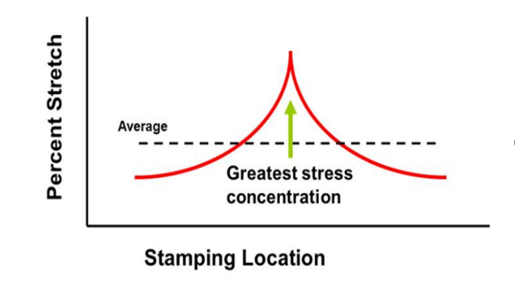
Figure 1: Peak strain in the localized area or embossment
Peak (concentrated) strains are susceptible to localized fractures when even slight variation exists in the forming process. Examples of variation include lubrication pattern and volume, die recipe including blank position, press conditions, and material characteristics.
A localized neck and/or fracture (Figure 2) reduces the sheet metal’s thickness, reducing part strength, and compromising functional performance such as fatigue life, crash worthiness, and stamping stiffness. There are a number of formability analysis tools that can differentiate localized and global fractures and enable die makers to implement die and process improvements that minimize fracture susceptibility. The result is a more robust stamping process.
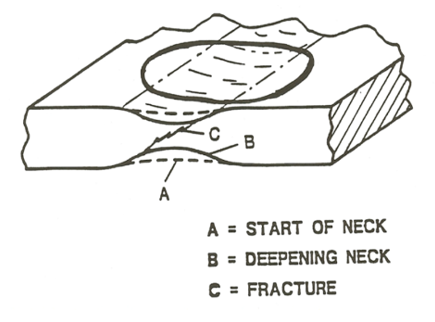
Figure 2: Schematic of Localized Necking and Fracture
Process control is critical; die recipe discipline is needed to minimize tinkering with die recipe, press settings, and lubrication settings. Mechanical properties of the sheet metal should be tracked to identify trends or variations in the material, and establish the material forming window. Typical mechanical properties that are available from the steel supplier are yield strength, tensile strength, n-value, total and uniformed elongation, and sheet thickness. Additional properties that should be determined include hole expansion and deep cup draw ratios. Failure to identify strain levels, process variables and variation will lead to a reactionary approach to controlling the output. This will lead to an increase in scrap, die-related downtime, and of course, costs.
Contributions made by Phoenix Group.
Blog, Joining, Joining Dissimilar Materials, main-blog, News, Resistance Spot Welding, RSW of Dissimilar Steel
This blog is a short summary of a published comprehensive research work titled: “Peculiar Roles of Nickel Diffusion in Intermetallic Compound Formation at the Dissimilar Metal Interface of Magnesium to Steel Spot Welds” Authored by Luke Walker, Carolin Fink, Colleen Hilla, Ying Lu, and Wei Zhang; Department of Materials Science and Engineering, The Ohio State University
*****
There is an increased need to join magnesium alloys to high-strength steels to create multi-material lightweight body structures for fuel-efficient vehicles. Lightweight vehicle structures are essential for not only improving the fuel economy of internal combustion engine automobiles but also increasing the driving range of electric vehicles by offsetting the weight of power systems like batteries.
To create these structures, lightweight metals, such as magnesium (Mg) alloys, have been incorporated into vehicle designs where they are joined to high strength steels. It is desirable to produce a metallurgical bond between Mg alloys and steels using welding. However, many dissimilar metal joints form intermetallic compounds (IMCs) that are detrimental to joint ductility and strength. Ultrasonic interlayered resistance spot welding (Ulti-RSW) is a newly developed process that has been used to create strong dissimilar joints between aluminum alloys and high-strength steels. It is a two-step process where the light metal (e.g., Al or Mg alloy) is first welded to an interlayer (or insert) material by ultrasonic spot welding (USW). Ultrasonic vibration removes surface oxides and other contaminates, producing metal-to-metal contact and, consequently, a metallurgical bond between the dissimilar metals. In the second step, the insert side of the light metal is welded to steel by the standard resistance spot welding (RSW) process.
Cross-section View Schematics of Ulti-RSW Process Development
For resistance spot welding of interlayered Mg to steel, the initial schedule attempted was a simple single pulse weld schedule that was based on what was used in our previous study for Ulti-RSW of aluminum alloy to steel . However, this single pulse weld schedule was unable to create a weld between the steel sheet and the insert when joining to Mg. Two alternative schedules were then attempted; both were aimed at increasing the heat generation at the steel-insert interface. The first alternative schedule utilized two current pulses with Pulse 1, high current displacing surface coating and oxides and Pulse 2 growing the nugget. The other pulsation schedule had two equal current pulses in terms of current and welding time.
Lap shear tensile testing was used to evaluate the joint strength using the stack-up schematically, shown below. Note the images of Mg and steel sides of a weld produced by Ulti-RSW.
Lap Shear Tensile Test Geometry and the Resultant Weld Nuggets
An example of a welded sample showed a distinct feature of the weld that is comprised of two nuggets separated by the insert: the steel nugget formed from the melting of steel and insert and the Mg nugget brazed onto the unmelted insert. This feature is the same as that of the Al-steel weld produced by Ulti-RSW in our previous work. Although the steel nugget has a smaller diameter than the Mg nugget, it is stronger than the latter, so the failure occurred on the Mg sheet side.
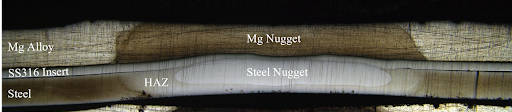
Joint strength depends on several factors, including base metal strength, sheet thickness, and nugget size, making it difficult to compare how strong a weld truly is from one process to another. To better compare the dissimilar joints created by different processes, joint efficiency, a “normalized” quantity was calculated for various processes used for dissimilar joining of Mg alloys to steels in the literature, and those results, along with the efficiencies of Ulti-RSW with inserts, are shown together below. Most of the literature studies also used AZ31 as the magnesium base metal. The ones with high joint efficiency (about 53%) in the literature are resistance element welding (REW) and friction stir spot welding (FSSW). In our study, Ulti-RSW with SS316 insert was able to reach an excellent joint efficiency of 71.3%, almost 20% higher than other processes.
Process Evaluation and Comparison
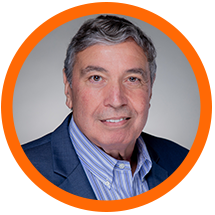
Thanks are given to Menachem Kimchi, Associate Professor-Practice, Dept of Materials Science, Ohio State University, and Technical Editor – Joining, AHSS Application Guidelines, for this article.
Blog, homepage-featured-top, main-blog, News
WorldAutoSteel is focused on advancing steel’s advantages in the automotive, autonomous vehicle, and future mobility industries. To encourage careers in engineering, we are committed to engaging with future engineers at post-secondary education organizations around the globe. Our most recent engineering project, Steel E-Motive, was created to help the industry meet the challenges of future mobility and Level-5 autonomous vehicles and eventually reach net zero emissions targets.
We engaged Ricardo plc to collaborate with our technical directors to develop a Level 5 Autonomous Vehicle for the Steel E-Motive project. The project uncovered a few challenges that were solved by student engineering teams through Senior Capstone Projects. Here we summarize the Side Door and Door Hinges project, created at Michigan Technological University by the engineering students and faculty members listed herein.
Michigan Tech University – Senior Capstone Project #2: Adaptation of SEM2 from People Mover to Commercial Delivery Vehicle
MTU Senior Capstone Team: Kyle Davis, Nick Palatka, Evan Larson, Logan Pietila, Blake Pietila, Tej Bergin
Introduction and Background
This Senior Capstone Design Team was sponsored by WorldAutoSteel and Ricardo Engineering (UK engineering and consultancy firm) to develop a solution for expanding the serviceability within a 24-hour period for Steel E-Motive 2 (SEM2), their extra-urban electric autonomous vehicle concept.
The SEM2 vehicle is a stretched 6-passenger commuter targeting longer journeys with expanded occupancy or additional luggage capacity. In non-commuting hours, the Mobility Fleet Operator would like to continue revenue generation by quickly adapting the vehicle for commercial delivery services. Currently, occupant packaging contrasts with the storage requirements of a package delivery vehicle; thus, the SEM2 vehicles can only be utilized to either transport people or goods. Our project aims to develop interior seating that enables quick removal and adaptation to an optimal delivery van.
Project Details and Results
From the requirements outlined by WorldAutoSteel, the team focused further research on interior vehicle and seat design. Modern delivery methods, delivery vehicle layout, passenger vehicle seat safety requirements, and seat folding or locking mechanisms were sub-categories of research that hold value within the project’s scope. The main takeaways from our research include different pin and slot mechanisms that are incorporated into a preliminary design for a quick-release system. We also benchmarked a vertical folding seat based on International Harvester designs, and we have modified these for application to the SEM2 vehicle’s specific needs.
The quick-release system will benefit MSP technicians responsible for performing the conversion of multiple SEM2 vehicles in its fleet at their depot during off-commuting hours. In under 30 minutes, the fleet operators (MSP) must be able to convert the vehicle from a vehicle stressing passenger comfort to an autonomous delivery van and vice versa, using common tools and techniques while meeting all necessary safety standards and regulations. The fleet operators that provide the robotaxi service are not expected to see any major disruption in ride services; however, they may observe improved utilization and profitability if they use the vehicles for package delivery.
Our current engineering requirements include:
- Maximum total payload of 675 kg (6 passengers and 6 seats)
- Individual seat weight of 30kg (6 seats)
- Total volumetric storage space requirement of 1 cubic meter
- Total seating width equal to or less than 1220 mm
- Changeover time less than 30 minutes and
- Minimal number of changeover movements (Fewer than 50 for the complete conversion from passenger to cargo transportation)
Concept Solutions
A graphical rendering of our selected system-level autonomous vehicle concept is provided in Figure 1. This design showcases two pairs of T-shaped rails placed in the fore-aft direction of the vehicle. These T-Rails are compatible with a slider system connecting to each seat leg’s bottom.
A sliced view of the rails and slider system are seen in Figure 2. On each slider, in the port-starboard direction, a circular slot approximately 20 mm in diameter (dependent on pin material and size) is cut-out to allow for the insertion of a spring-loaded steel pin. This pin engages both the slider and an equivalent slot cut into the rail, to allow the seat to be locked into a specified position along the rail (Figure 3). The rails run the full length of the vehicle’s interior, allowing 3 seating modules to be placed and locked into a position. Inserting and removing the slider on the rails will be possible through narrow sections where the slider can be vertically lifted or placed on the rail system.
In order to “drop the seats” onto the rails without manual lifting, the team has designed an accompanying “pallet jack accessory” that will be able to hold, transport, and lower the seating modules onto the rail system through the use of an industry-standard pallet jack with a lifting range of 6 inches. The pallet jack accessory can be seen in Figure 1, item C.
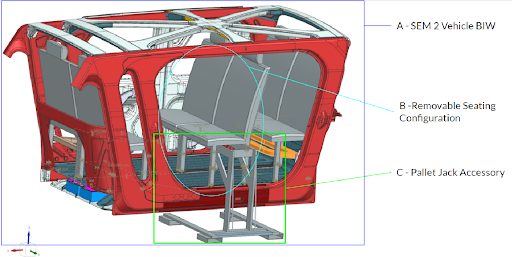
Figure 1 – System level concept – rapid adaptation of SEM2 to autonomous delivery services
The slider mechanism, seen in Figure 2 below, will house a ball roller bearing that allows for the translational motion along the rail to slide the seats into position.
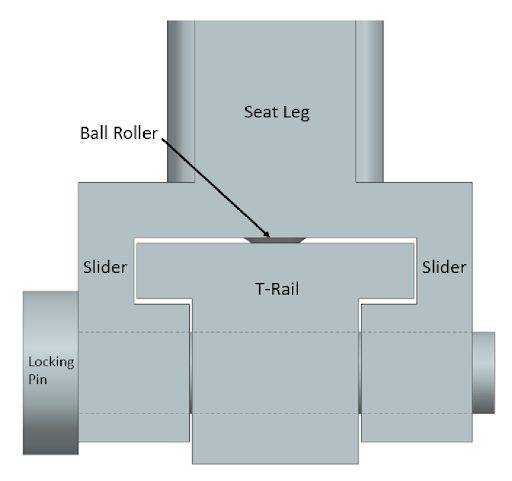
Figure 2 – Slider mechanism in Steel E-T-rail system
The roller bearing bolts into the slider mechanism allowing for fast and easy replacement. The T-Rails are then bolted to the structural members of the vehicle, where engineers from WorldAutoSteel have confidence the design can withstand any and all static and dynamic loading scenarios. The rails will feature a narrow section near the center, allowing the seating module to be removed. This narrow section is tapered to allow the slider to be “homed” and slid into its final, fixed position.
The arms of the pallet jack system reach out to allow a set of seats to be placed on the rail at one time. The pallet jack will drive through the open slots on the ground, lift the seats to the vehicle, align itself using markings on the vehicle, and drop the seats into the rails, where they can then be manually moved to their correct position. The system is designed to remove and insert these seats as fast as possible while exerting minimum effort that might stress the MSP technician. With ease of use and safety being the critical elements of every project, we’ve removed manual lifting from the equation and ensured a factor of safety of 2 is kept for all required crash loads under our current design.
Many integral components are COTS parts and can be bought in bulk to use for mass production as well as reserved parts, helping maintain low cost of ownership for the MSP. The components that are not COTS items, such as the rails and sliders, can be manufactured using high-volume, low-cost fabrication techniques such as stamping. Assembly of the system will be just as easy as all components are connected together using industry-standard fastening and welding techniques.
Conclusions
Validation – To confirm the ease of changeover and our objectives, a simulation was conducted to estimate the time and difficulty of changing from delivery service to people transporter. In a warehouse setting, a location was established as the “vehicle maintenance spot.” We developed a “seat module storage area” approximately 30 meters away. In this simulation, the following steps were conducted:
- A pallet jack was pre-staged near the vehicle, signaling the beginning of the conversion and timer
- A technician walked 30 m to the seating storage area and picked up the seats via accessory
- They carried seats back to the vehicle, aligned the pallet jack with the door, lowered seats onto the rail system, and removed the pallet jack accessory from the vehicle
- They slid the seats into the correct position and inserted the locking pin into its slot
This simulation was repeated twice to replicate the insertion of all six seats. To remove the seats, the steps would be reversed. After five runs of this simulation, ensuring adequate time to perform each simulated step, the average time to complete the simulation was 5 minutes 17 seconds with a total of 24 required movements.
These values were well within the 30-minute and 50-movement objectives. For further validation, we’ll repeat these simulations in the opposite direction, ie, removing the seats to transform into the delivery van. Finally, in Phase 2 of this project, we’ll continue to evaluate seat frame/track componentry to ensure robustness and durability in the proposed solutions.
More Info About Steel E-Motive
We are grateful to our student teams, their supportive leaders, and the universities providing automotive engineering education to our future industry leaders. Their contributions to Steel EMotive have been invaluable.
Interested in learning more about Steel E-Motive and the infinite tunability of steel for Future Mobility? Download the full engineering report here: Steel E-Motive Engineering Report
Blog, homepage-featured-top, main-blog, News
As an organization focused on advancing the advantages of steel to the automotive and future mobility industries, WorldAutoSteel is committed to engaging with future engineers at universities and colleges around the globe.
Our most recent engineering project, Steel E-Motive, was designed to unveil and meet the challenges of future autonomous vehicles that will help the automotive industry reach net zero emissions targets.
For the Steel E-Motive project, we engaged Ricardo plc to collaborate with our technical directors to develop a Level 5 Autonomous Vehicle. The project uncovered a few challenges that were solved by student engineering teams through Senior Capstone Projects.
Here we summarize the Side Door and Door Hinges project, created at Michigan Technological University by the students and faculty members listed herein.
Steel E-Motive Side Door Functionality and Door Hinge Assessment
The MTU Senior Capstone Team members were Gavin Sheffer, Leander Daavettila, Rob Oestreich, Steven Turnbull, Andrew Mitteer, and Jesse Ebenhoeh.
Introduction and Background
The MTU Senior Capstone Design Team, sponsored by WorldAutoSteel and the Auto/Steel Partnership, was challenged to design a new door hinge for the Steel E-Motive side closure mechanism. The current hinge design was referenced, but a few operational issues were identified for this team’s assessment and engineering study.
The door had unconstrained degrees of freedom, allowing it to swing freely about one axis. Thus, the project included a review of the kinematics of the door opening and hinge design for attachment to the A and C pillars.
The previous hinge design interferes with the all-wheel steering, meaning in emergency situations, passengers could get trapped in the vehicle. For SEM1, it was also observed that when the wheels are turned, they would block the doors from opening fully, constraining the passenger exit (Figure 1 below).
An emergency release mechanism was needed to allow users to escape if a crash or electrical failure prevented the doors from opening.
Power requirements and electric motor sizing for the hinge mechanism needed to be defined.
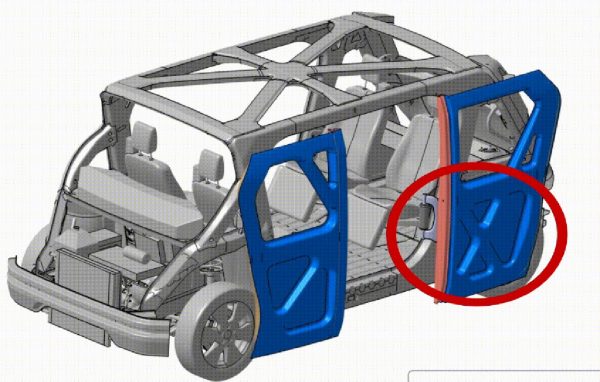
Figure 1: Side door opening constrained by present hinge mechanism and wheel position.
Project Details and Results
MTU’s design solution uses a four-bar linkage hinge design to keep the door parallel to the vehicle’s body to avoid damage to either the door or the body. The team used a 4:1 gear ratio for the drive motor to open the door. Finally, one of the pins in the secondary arm linkage is accessible by passengers and removable, allowing users to manually push the door open in the event of an emergency (see Figure 2).
Their solution includes pressing a button to open and close the door. The stepper motor receives the input from the button and rotates the gears; the gears then rotate the primary arm, which drives the door. The primary arm is mounted to the door in two locations with bolts. The secondary arm is added to the mechanism to create a four-bar linkage, which helps maintain door orientation during operation. An emergency release was designed and added to the secondary arm to release the four-bar linkage. This allows the door to swivel around a turned wheel in the event of an emergency.
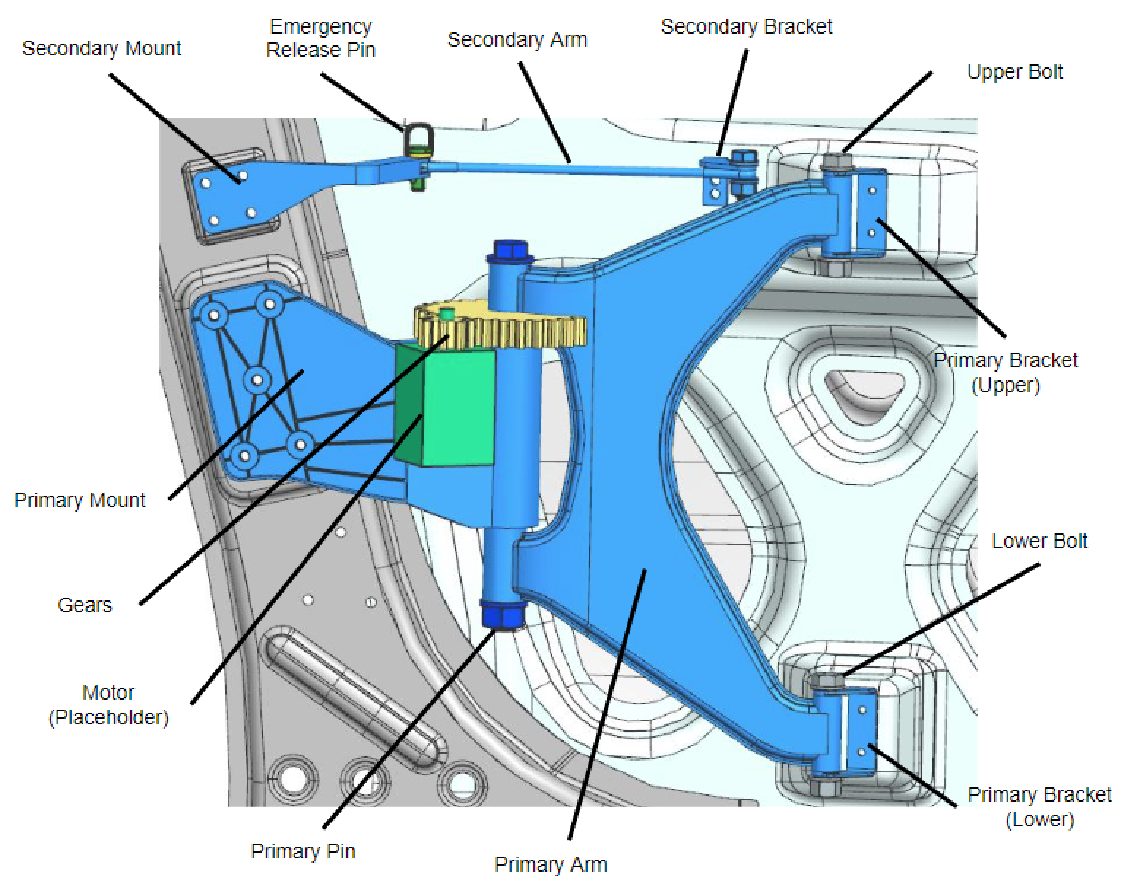
Figure 2: 3D model of the final design less the gear cover for clarity.
The emergency release was designed for safety and manufacturability. The emergency release pin was simplified to utilize off-the-shelf pins. A relay is suggested to cut power to the motor and allow the door to be manually pushed open. A gear cover was added for safety to protect the occupants from getting pinched by the gears. To maintain and service the design, each assembly part was designed to be attached with threaded fasteners. A gear drive was created so we could use a more cost-efficient motor. The gear ratio decreased the amount of torque required. The material was chosen based on strength and sustainability.
Conclusions
The CAD geometry and the quarter-scale prototype met all of our engineering requirements and objectives. The CAD model defines the mass and emergency release mechanism, and the kinematics are verified by the quarter-scale prototype. The FEA simulation verified set and sag under normal and abusive loading conditions.
In identifying specifications required for the full model, the projected production cost for one mechanism is $471. This includes stamped and cast components, off-the-shelf components such as the stepper motor and fasteners, and the assembly cost.
The team compared using AHSS for the components as opposed to an aluminum alloy. Steel components are stronger, half the price of aluminum, and produce 1/3 of the carbon emissions compared to the same amount of aluminum.
The prototype was 3D printed from PLA plastic at a quarter scale. This serves as a model to be shown by Auto/Steel Partnership for future presentations. It was also used to verify the kinematics of the door motion.
Learn more about Steel E-Motive and download the FREE Engineering Report here: Steel E-Motive