AHSS, Blog, homepage-featured-top, Joining, main-blog, News, Resistance Spot Welding, RSW of Dissimilar Steel, Steel Grades, Tool & Die Professionals
Urbanization and waning interest in vehicle ownership point to new transport opportunities in megacities around the world. Mobility as a Service (MaaS) – characterized by autonomous, ride-sharing-friendly EVs – can be the comfortable, economical, sustainable transport solution of choice thanks to the benefits that today’s steel offers.
The WorldAutoSteel organization is working on the Steel E-Motive program, which delivers autonomous ride-sharing vehicle concepts enabled by Advanced High-Strength Steel (AHSS) products and technologies.
The Body structure design for this vehicle is shown in Figure 1. It also indicates the specific joint configuration of 5 layers AHSS sheet stack-up as shown in Table 1. Resistance spot welding parameters were developed to allow this joint to be made by a single weld. (The previous solution for this welded joint is to create one spot weld with the bottom 3 sheets indicated in the table and a second weld to join the top 2 sheets, combining the two-layer groups to 5T stack-up.)
NOTE: Click this link to read a previous AHSS Insights blog that summarizes development work and recommendations for resistance spot welding 3T and 4T AHSS stack-ups: https://bit.ly/42Alib8
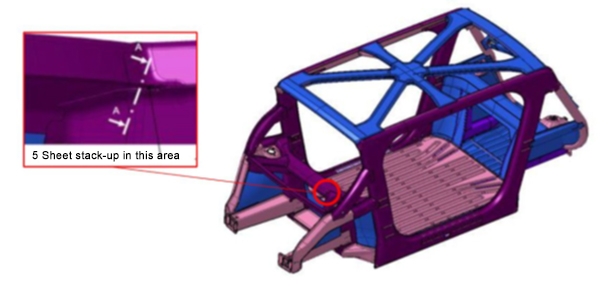
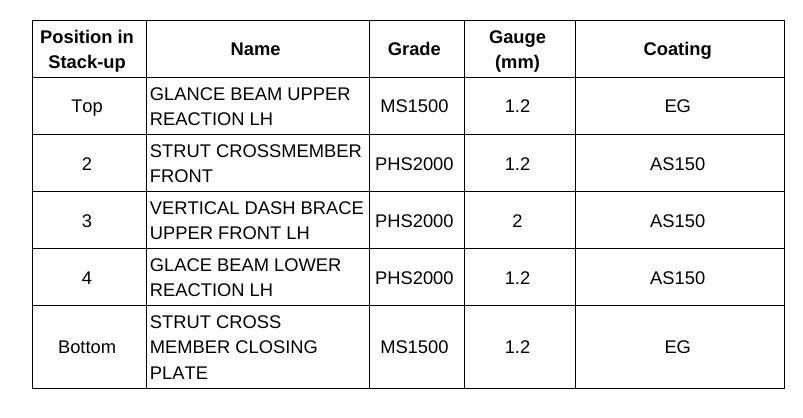
Table 1. Provided materials organized in stack-up formation showing part number, name, grade, gauge in mm, and coating type. Total thickness = 6.8 mm
The same approach of utilizing multiple current pulses with short cool time in between the pulses was shown to be most effective in this case of 5T stack-up. It is important to note that in some cases, the application of a secondary force was shown to be beneficial, however, it was not used in this example.
To establish initial welding parameters simulations were conducted using the Simufact software by Hexagon. As shown in Figure 2, the final setup included a set of welding electrodes that clamped the 5-layer AHSS stack-up. Several simulations were created with a designated set of welding parameters of current, time, number of pulses, and electrode force.
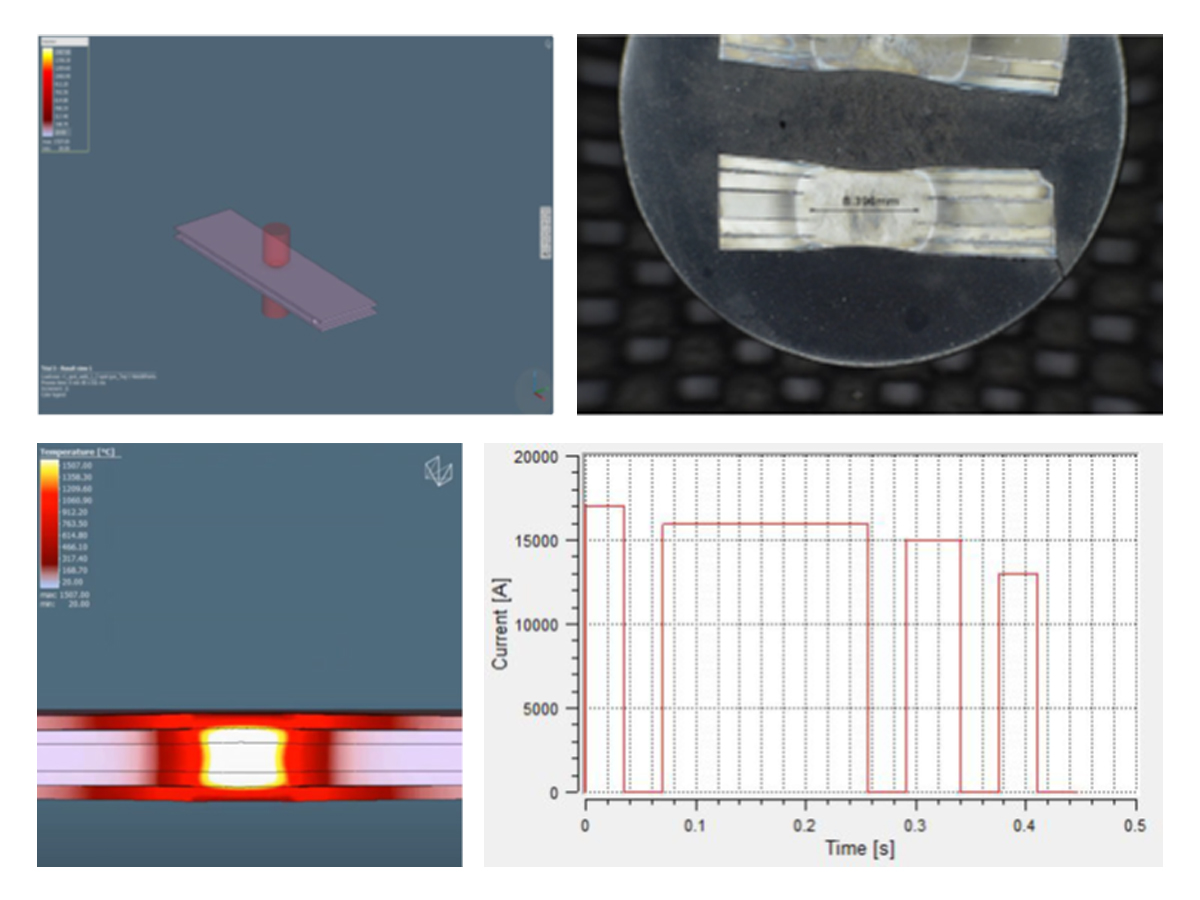
Figure 2. Example of simulation and experimental results showing acceptable 5T resistance spot weld (Meets AWS Automotive specifications)
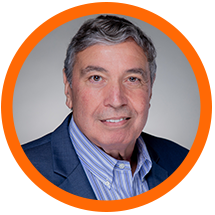
Thanks is given to Menachem Kimchi, Associate Professor-Practice, Dept of Materials Science, Ohio State University and Technical Editor – Joining, AHSS Application Guidelines, for this article.
1stGen AHSS, 2ndGen AHSS, 3rdGen AHSS, AHSS, Blog, homepage-featured-top, main-blog, News, Production Managers, Steel Grades, Tool & Die Professionals
This month’s blog was contributed by Peter Ulintz, Precision Metalforming Association. This content originally appeared in the September 2023 issue of MetalForming Magazine under the title “Stronger AHSS Knowledge Required for Metal Stampers” and has been reproduced with the permission of MetalForming Magazine.
Metal stampers and die shops experienced with mild and HSLA steels often have problems making parts from AHSS grades. The higher initial yield strengths and increased work hardening of these steels can require as much as four times the working loads of mild steel. Some AHSS grades also have hardness levels approaching the dies used to form them.
Dies Get Tougher
Metal stampers and die shops experienced with mild and HSLA steels often have problems making parts from AHSS grades. The higher initial yield strengths and increased work hardening of these steels can require as much as four times the working loads of mild steel. Some AHSS grades also have hardness levels approaching the dies used to form them.
The higher stresses required to penetrate higher-strength materials require increased punch-to-die clearances compared to mild steels and HSLA grades. Why? This clearance acts as leverage to bend and break the slug out of the sheet metal. Stronger materials need longer levers to bend the slug. The required clearance is a function of the steel grade and tensile strength, and sheet thickness.
Increasing cutting clearance can result in punch cracking and head breakage due to higher snapthrough loads and reverse-unloading forces within the die. Adding shear angles to the punch face helps reduce punch forces and reverse unloading.
Tight-cutting clearances increase the tendency for die galling and chipping. The severity of galling depends on the surface finish and microstructure of both the tool steel and work material. Chipping can occur when process stresses are high enough to cause low-cycle fatigue of the tooling material, indicating that the material lacks toughness.
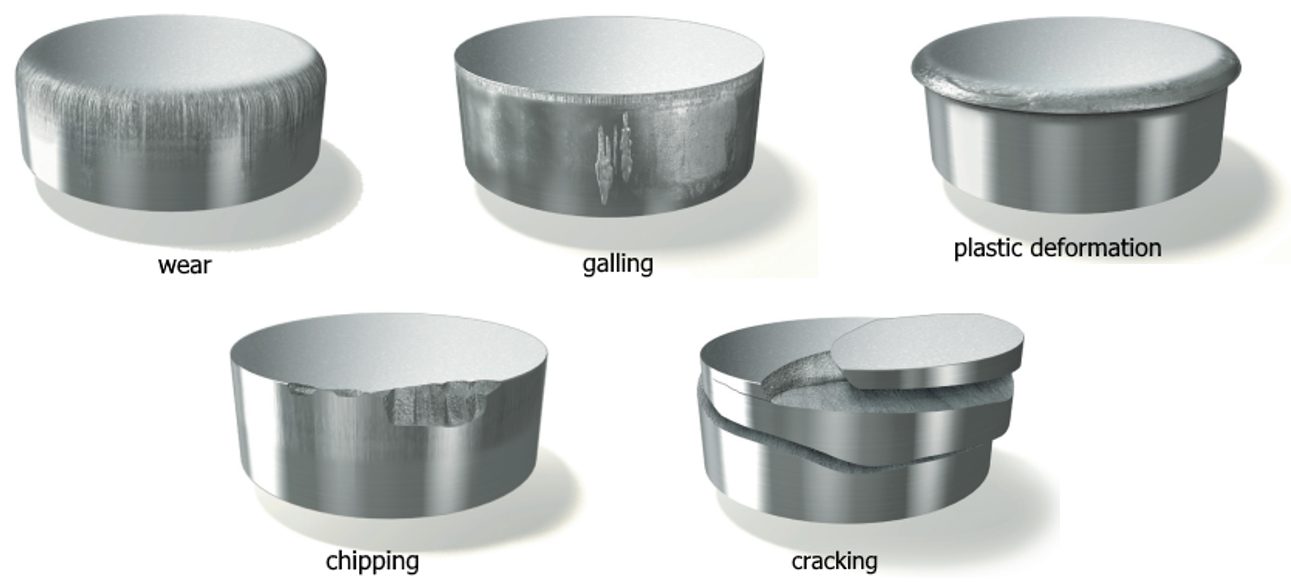
Stamping Tool Failure Modes (Citations T-20 and U-7)
Tempering of tools and dies represents a critical heat-treatment step and serves more than one purpose, but of primary concern is the need to relieve residual stresses and impart toughness. Dies placed in service without proper tempering likely will experience early failure.
Dies made from the higher-alloy tool-steel grades (D, M or T grades) require more than one tempering step. These grades contain large amounts of retained austenite and untempered martensite after the first tempering step and require at least one more temper to relieve internal stresses, and sometimes a third temper for even greater toughness.
Unfortunately, heat treatment remains a “black-box” process for most die shops and manufacturing companies, which send soft die details to the local heat treat facility, with hardened details returned. A cursory Rockwell hardness test may be conducted at the die shop when the parts return. If they meet hardness requirements, the parts usually are accepted, regardless of how they may have been processed—a problem, as hardness alone does not adequately measure impact toughness.
Machines Get Stronger
The increased forces needed to form, cut and trim higher-strength steels create significant challenges for pressroom equipment and tooling. These include excessive tooling deflections, damaging tipping-moments, and amplified vibrations and snapthrough forces that can shock and break dies—and sometimes presses. Stamping AHSS materials can affect the size, strength, power and overall configuration of every major piece of the press line, including material-handling equipment, coil straighteners, feed systems and presses.
Here is what every stamper should know about higher-strength materials:
- Because higher-strength steels require more stress to deform, additional servo motor power and torque capability may be needed to pull the coil material through the straightener. Additional back tension between the coil feed and straightening equipment also may be required due to the higher yield strength of the material in the loop as the material tries to push back against the straightener and feed system.
- Higher-strength materials, due to their greater yield strengths, have a greater tendency to retain coil set. This requires greater horsepower to straighten the material to an acceptable level of flatness. Straightening higher-strength coils requires larger-diameter rolls and wider roll spacing in order to work the stronger material more effectively. But increasing roll diameter and center distances on straighteners to accommodate higher-strength steels limits the range of materials that can effectively be straightened. A straightener capable of processing 600-mm-wide coils to 10 mm thick in mild steel may still straighten 1.5-mm-thick material successfully. But a straightener sized to run the same width and thickness of DP steel might only be capable of straightening 2.5 mm or 3.0-mm thick mild steel. This limitation is primarily due to the larger rolls and broadly spaced centers necessary to run AHSS materials. The larger rolls, journals and broader center distances safeguard the straightener from potential damage caused by the higher stresses.
- Because higher-strength materials require greater stress to blank and punch as compared to HSLA or mild steel, they generate proportionally increased snapthrough and reverse-unloading forces. High-tensile snapthrough forces introduce large downward accelerations to the upper die half. These forces work to separate the upper die from the bottom of the ram on every stroke. Insufficient die-clamping force could cause the upper-die half to separate from the bottom of the ram on each stroke, causing fatigue to the upper-die mounting fasteners.
- Because energy is expended with each stroke of the press—and this energy must be replaced—critical attention must focus on the size (horsepower) of the main drive motor and the rotational speed of the flywheel in higher-strength-steel applications. The main motor, with its electrical connection, provides the only source of energy for the press and it must generate sufficient power to meet the demands of the stamping operation. The motor must be properly sized to replace the increased energy expended during each press stroke. For these reasons, some stampers consider the benefits of servo-driven presses for these applications.
As steels becomes stronger, a corresponding increase in process knowledge is required in terms of die design, construction and maintenance, and equipment selection.
You can read more about these topics at these links:
Tooling and Die Wear
Coil Processing Straightening and Leveling
Press Requirements
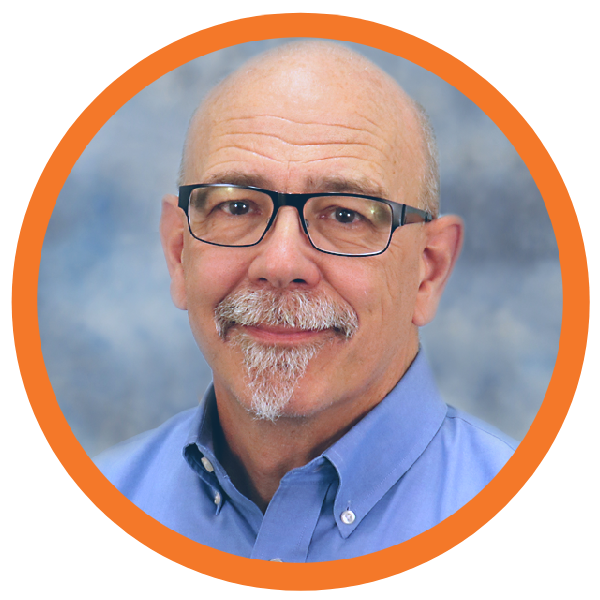
Thanks go to Peter Ulintz, of the Precision Metalforming Association (PMA) for authoring this article. Ulintz was employed in the metal stamping and tool & die industries for 38 years before joining Precision Metalforming Association (PMA) in 2015. He provides industry-related training and seminars in Stamping Press Operation and Setup; Designing and Building Metal Stamping Dies; Die Maintenance and Troubleshooting; Metal Stamping Design for Manufacturability; Deep Draw Tooling and Process Technology; Stamping Higher Strength Steels; and Problem Solving in the Press Shop. Peter is a contributor to ASM Handbook, Volume 14B, Metalworking: Sheet Forming (2006) and writes the monthly column, Tooling by Design, for PMA’s monthly publication, MetalForming Magazine.
AHSS, Blog, main-blog, News
Net Zero Emissions by 2050 – it’s a goal for future mobility that can seem distant and daunting. But over the past five years, WorldAutoSteel’s global automotive steel suppliers have conducted extensive research that illuminates a path forward. The Steel E-Motive concept – borne of this research – can be a catalyst for reaching the Net Zero goal.
Urbanization and changing attitudes towards vehicle ownership point to new transport opportunities in megacities worldwide. Mobility as a Service (MaaS) – characterized by autonomous, ride-sharing-friendly EVs – can be the comfortable, economical, and sustainable transportation solution of choice thanks to the benefits that modern steels offer, which will foster the higher vehicle occupancy that is critical to Net Zero ambitions.
Here, we break down the many benefits of the Steel E-Motive vehicle.
The Key Steel E-Motive Vehicle Features for Future Mobility
The Steel E-Motive Vehicle features seven key Advanced High-Strength Steel structural innovations to create a safe, economical vehicle.
- A B-Pillarless open-body structure offers excellent comfort, accessibility and easy ingress/egress.
- The Short Front Crash Zone design meets all global high-speed frontal crash requirements.
- The AHSS Extended Front Passenger Protection Zone provides excellent cabin intrusion protection for occupants.
- The Small Offset Crash Glance Beam minimizes the energy pulse into the occupant cabin, reducing the potential for passenger injuries.
- Hex beam energy absorbers provide superior battery protection for both side pole and deformable barrier crashes.
- The Scissor Door with Virtual B-Pillars offers excellent passenger visibility while saving mass and costs.
- The Coverless Battery Carrier Frame concept rewards 37% mass savings over benchmarks and 27% cost reduction; it also affords enhanced battery protection from road debris and other floor impacts.
The Steel E-Motive vehicle is created to meet Level 5 autonomy, meaning it is void of driver interfaces and does not require any human attention. With all of these features and more, the SEM architecture affords a spacious, safe, and comfortable cabin for occupants.
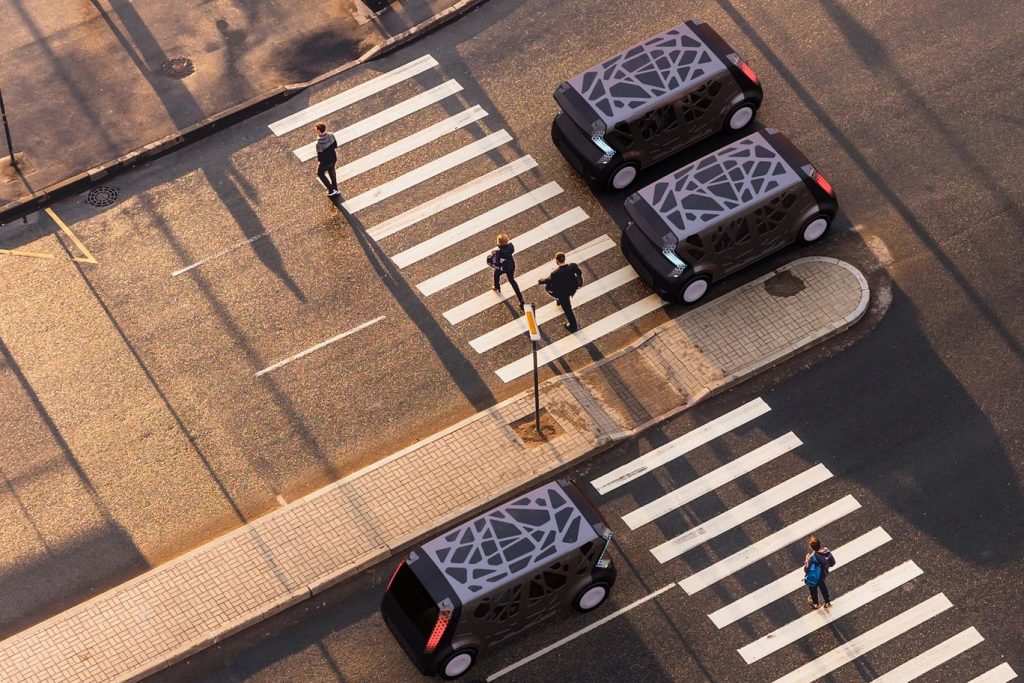
Steel E-Motive concepts are designed to help pave the way to a Net Zero future.
Exceeds Crash Guidelines
The Steel E-Motive vehicle is one of the world’s first autonomous vehicle concepts to validate and report excellent performance measured against the most stringent global crash requirements, which aligns with an IIHS “Good” rating. Modern Advanced High-Strength Steel product and fabrication process innovations enable the vehicle design to exceed these stringent crashworthiness standards while minimizing overall mass and production emissions.
Created to Be Affordable
Considering both production and life cycle costs, Steel E-Motive concepts have low maintenance requirements and are designed to be manufacturable using the world’s global manufacturing infrastructure at costs that support profitable margins, both for the vehicle manufacturer and the mobility service providers. Steel E-Motive is a fully engineered vehicle program that start-up companies can use to significantly reduce their cost and time to market.
Designed with Sustainability in Mind
The viability of any MaaS disrupter is contingent on cost competitiveness versus existing solutions, such as private ownership or taxis.
Moreover, our designs minimize steel thicknesses for lower mass while maximizing material utilization for lower steel production and emissions. Overall, the vehicle design offers the potential for ~86% CO2 emissions reduction when all factors contributing to sustainability are optimized. Autonomy further reduces operating emissions due to drive cycle smoothing.
To achieve our Net Zero future, high-occupancy vehicle usage is crucial and must be appealing for riders and profitable for providers.
Steel E-Motive concepts play a vital role in enabling Future Mobility Solutions THAT ONLY STEEL CAN MAKE REAL. Learn more about the program: https://steelemotive.world/
3rdGen AHSS, AHSS, Blog, General, main-blog, Metallurgy, News, Steel Grades
The Steel E-Motive program–commissioned by WorldAutoSteel in partnership with Ricardo plc–has developed the world’s first fully autonomous electric vehicle body structure concept purpose-fit for ride-sharing. This global steel industry initiative showcases the strength and durability of steel with an eye on playing a pivotal role in reaching net zero emissions targets.
Download the Steel E-Motive Engineering Report
Here, we break down the many benefits of the Steel E-Motive concept that only Advanced High-Strength Steel (AHSS) can enable.
Steel E-Motive Was Conceived as a Level 5 Autonomous Vehicle
The Steel E-Motive concept is designed to be a Level 5 autonomous vehicle, so it does not include any driver interfaces. The design features a spacious, airy cabin with rear-facing front-passenger seat configurations. The B-pillarless structure and unique battery system design offer easy ingress and egress.
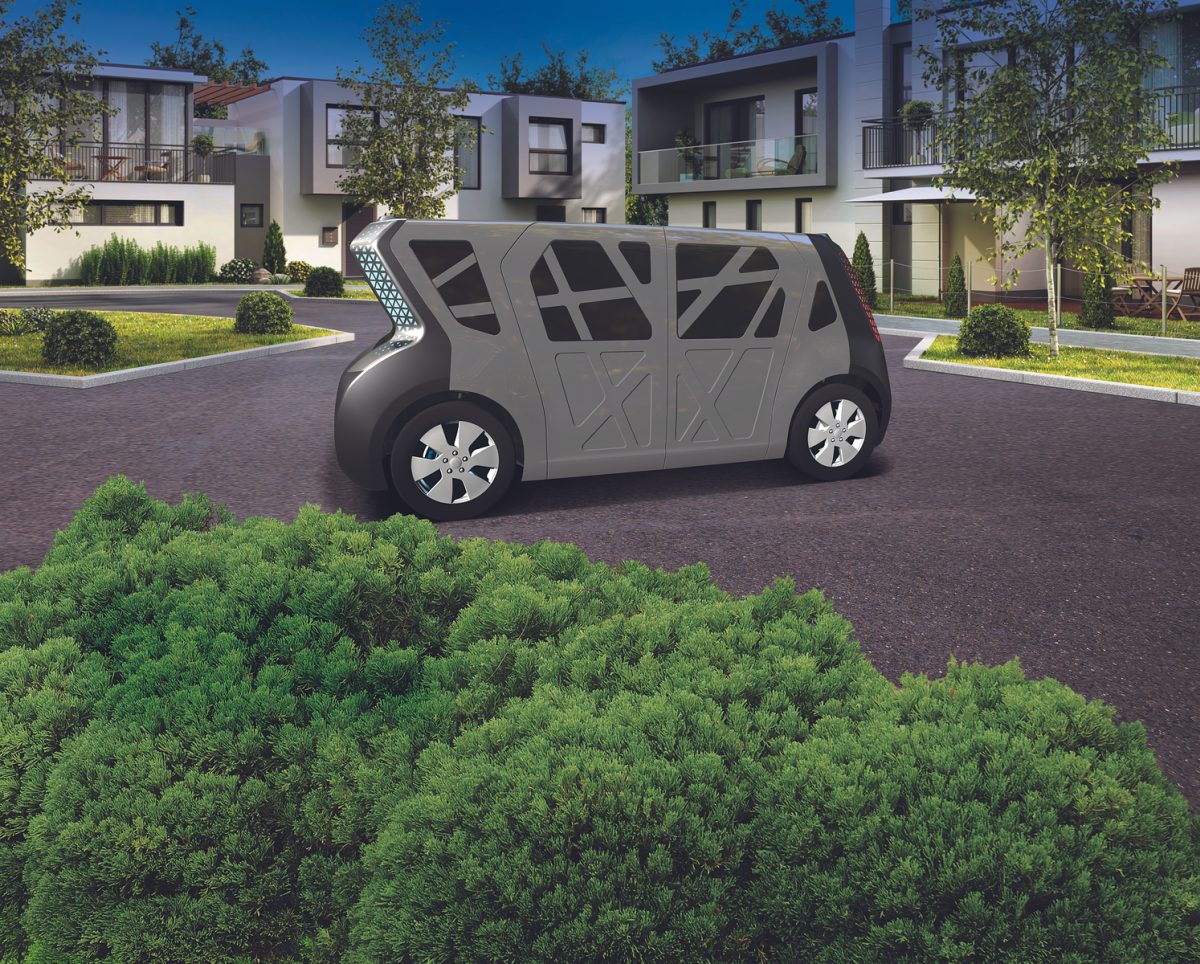
The Steel E-Motive concept is designed to be a Level 5 autonomous vehicle.
Designed to Exceed Future Mobility Safety Standards
Modern Advanced High-Strength Steels innovations allow the Steel E-Motive autonomous vehicle to exceed current global high-speed crashworthiness standards. By using AHSS, the Steel E-Motive vehicle is the first to acknowledge compliance with NHTSA and IIHS safety standards publicly.
For example, the 4-passenger B-sized urban concept SEM1 introduced a new front-end passenger protection zone. This design features the small overlap Glance Beam, which forces the car to “glance” off the barrier and reduces passenger cabin intrusion. It also lowers the crash pulse and ultimately minimizes passenger injury. Advanced High-Strength Steels also offer strong battery protection and preserve door ring integrity in this autonomous vehicle.
The Evolution of Advanced High-Strength Steel
Over the past quarter century, vehicle concept projects have showcased the continuous advancement of steel. In 1998, global steelmakers introduced the Ultralight Steel Auto Body, which used one of the earliest forms of AHSS. This project demonstrated steel’s ability to reduce weight without compromising safety.
By 2010, we introduced the Future Steel Vehicle concept. Using 27 AHSS materials, the body structure design reduced mass by over 35%. Steel materials enable these massive reductions while allowing the design to meet global crash and durability requirements.
The Steel E-Motive concepts benefit from no fewer than 64 materials under the AHSS umbrella. The “infinite tunability” of AHSS allows product customization by designers and engineers to select exactly the right steel for every need and purpose in the vehicle.
Key Attributes of the Steel E-Motive Autonomous Vehicle
From lowering the carbon footprint to massively reducing weight, the Steel E-Motive vehicle offers first-of-its-kind benefits for future mobility made possible by AHSS.
Steel allows the vehicle to reduce weight without sacrificing strength. For example, 66% of the Steel E-Motive autonomous vehicle structures’ materials have an Ultimate Tensile Strength of at least 1,000 MPa, and these materials’ weighted average tensile strength is 1259 MPa.
By using 33% Press Hardened Steels and 11% 3rd Generation AHSS, the design includes complex geometries fully formed by hot and cold-stamped gigapascal steels.
In another example, 43% of the Steel E-Motive structure is fabricated from material-efficient processes such as press hardening, hydroforming, roll forming, and roll stamping. With these processes, the steel body design maximizes material utilization and minimizes scrap rate. This means less material is produced, lowering the structure’s carbon footprint. These achievements reduce manufacturing costs to support a profitable margin both for the vehicle manufacturer and the mobility service provider.
Using AHSS, the Steel E-Motive autonomous vehicle’s body structure mass is 25% lower than benchmark vehicles of a similar volumetric footprint. Additionally, Steel E-Motive realizes a 27% lower battery frame cost than a fully enclosed battery design, with 37% mass savings.
In conclusion, the Steel E-Motive program stands as a remarkable testament to the innovative potential of steel in shaping the future of mobility and autonomous vehicles. With its groundbreaking design, the Steel E-Motive concept paves the way for Level 5 autonomous electric vehicles prioritizing safety, sustainability, and efficiency.
Harnessing the unique attributes of AHSS, this global steel industry initiative also showcases the remarkable evolution of steel materials over the years. From Ultralight Steel Auto Body to Future Steel Vehicle, the journey of AHSS has been one of continuous improvement, leading to Steel E-Motive’s exceptional achievements in weight reduction, enhanced safety, and minimized environmental impact.
As we venture into an era of net-zero emissions and advanced mobility solutions, the Steel E-Motive concept proudly positions steel as a driving force in shaping a cleaner, safer, and more connected future.
Download the Steel E-Motive Engineering Report
Blog, homepage-featured-top, main-blog, News
WorldAutoSteel has a 30-year legacy of steel demonstration all the way back to the Ultra-Light Steel Auto Body (ULSAB), whose engineering report is still being downloaded from our worldautosteel.org site today. The one you may remember best is the FutureSteelVehicle (FSV), results of which we launched in 2011. FSV demonstrated steel innovation for not only Battery Electric vehicles (BEV) but also Fuel Cell vehicles (FCV). Steel E-Motive is the sixth of our global steel industry programs.
So Why Mobility as a Service?
The Automotive sector is undergoing the most rapid change in 40 years. This transformation shifts our thinking – from the movement of vehicles to the efficient movement of people and goods. Over the past eight years, we have conducted extensive research into global trends such as urbanization, transport emissions reduction, as well as the waning interest in vehicle ownership among the young and old. This is especially prevalent in megacities characterized by pollution, congestion, limited parking and enormous ownership costs. Our research concluded that mobility as a service (MaaS) will grow exponentially in high population areas and would place a significant challenge on vehicle design and manufacturing. Therefore, we needed to make sure we as an industry were active and visible in providing STEEL solutions in this new market place.
Steel E-Motive will demonstrate the benefits of steel, linking the properties of the material to the required architectures and attributes for MaaS vehicles.
This program will demonstrate the benefits of steel, linking the properties of the material to the required architectures and attributes for MaaS vehicles. It connects us with original equipment manufacturers (OEMs) and future mobility providers (FMPs), reinforcing steel’s advantages in strength, durability, sustainability and affordability.
An autonomous BEV structure aligns perfectly with steel’s best attributes, however most new concepts trial alternative materials. The global steel industry is investing significantly in product and fabrication development to continually prepare for the next challenge. High Strength and Advanced High-Strength Steel (AHSS) portfolios have grown from the 11 highlighted in the ULSAB program, to more than 60 grades available for use in designing and optimizing Steel E-Motive’s autonomous BEV architecture. Third Generation AHSS (3rd Gen AHSS) will have a prominent role in Steel E-Motive’s body-in-white, taking strength levels ever higher while improving manufacturability. And our industry continues to evolve Press Hardened Steels (PHS) with strength levels upwards of 2000 MPa.
Finally, efficient fabrication processes such as roll stamping, press hardening, and hydroforming use less steel and therefore contribute lower vehicle production emissions. These are the details being highlighted in Steel E-Motive, where we hope to demonstrate that only Steel can make it Real.
Steel E-Motive: A game changing, world first?
Many OEM’s and mobility service providers follow the typical vehicle development process where they adapt an existing vehicle structure to the new vehicle requirements. We don’t have that in Steel E-Motive We believe Steel E-Motive is one of the world’s firsts.
- The first for a Level 5 autonomous vehicle that is compliant with global high-speed crash requirements.
- The first autonomous vehicle to be a conventional high-volume stamped steel body construction, creating an affordable platform for the mobility service provider.
- First to offer a competitive, robust, and sustainable MaaS solution.
For engineers, being first is very exciting but a little nerve wracking – there are no benchmarks out there. There is less to “hang on to.” We’re on our own. Target setting is more challenging; we are the benchmark. Time will tell if we make it to the automotive hall of fame.
We are producing concepts for two BEVs based on a single modular platform. SEM1 (Figure 1) is a front-wheel drive short wheelbase urban version for inter-city travel for four passengers. It has a compact design and vehicle footprint, comparable in footprint to a European B/C segment size. SEM2 (Figure 2) is an all-wheel drive, long wheelbase extra urban version designed to carry up to six passengers. It has an adaptable interior volume that can result in additional luggage capacity compared to SEM1.

Figure 1: SEM1 Vehicle Specifications (© WorldAutoSteel 2022)
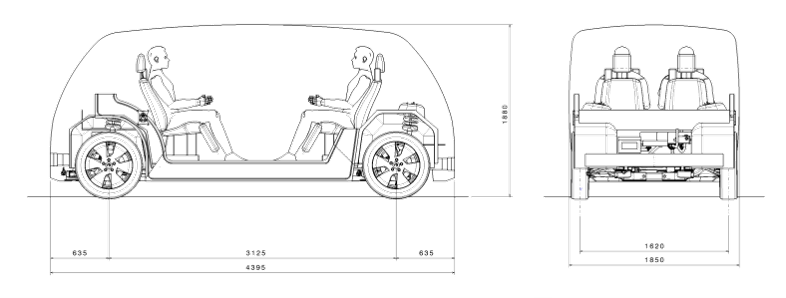
Figure 2: SEM2 Vehicle Specifications (© WorldAutoSteel 2022)
Body in White Steel Usage
Steel E-Motive benefits from a broad portfolio of steel grades and fabrication process, as identified by our member steel experts. The design is nearly finalized, and material selections are being evaluated against various performance targets with the representative structure shown in Figure 3 with high PHS usage at this stage in the design (as of May 2022). This is mainly driven by the safety requirements. Steel E-Motive BIW steel and steel technologies include:
- Right steel grade in the right place
- Significant proportion of >1500MPa grades, primarily for occupant and battery intrusion zones
- Mixture of stamped, roll formed, roll stamped, press hardened steel and hydroformed parts
- Spotweld, laser weld and structural adhesive

Figure 3: Steel E-Motive’s Body-in-White Steel usage as of May 2022. (© WorldAutoSteel 2022)
At the Core of the Steel E-Motive Concept Is an Innovative Battery Design
Figure 4 shows Steel E-Motive’s battery frame design’s construction:
- Battery modules and cooling plates are mounted to an AHSS carrier frame (off-line).
- The carrier frame is mounted to the body structure (in general assembly).
- The BIW floor acts as the top cover and provides sealing.
- The AHSS bottom cover plate provides impact protection.
This design provides significant cost and weight savings, as well as improved NVH. This extremely efficient package does not compromise safety and enables a flat floor with a lower step-in height.
Figure 4: Steel E-Motive Battery package assembly. (© WorldAutoSteel 2022)
Competitive Body Stiffness with an Open B-Pillarless Body Structure
With clean sheet design, and generally less package constraints in a Level 5 vehicle, our design teams have had more freedom to engineer and optimize the crash and stiffness structural loadpaths. We used topology, optimization, and Virtual Reality tools to determine the most efficient structural loadpaths (Figure 5). The results informed the joint designs and enabled optimization of the joining and structural adhesives. These steps and the advantage of steel’s high modulus resulted in impressive performance.
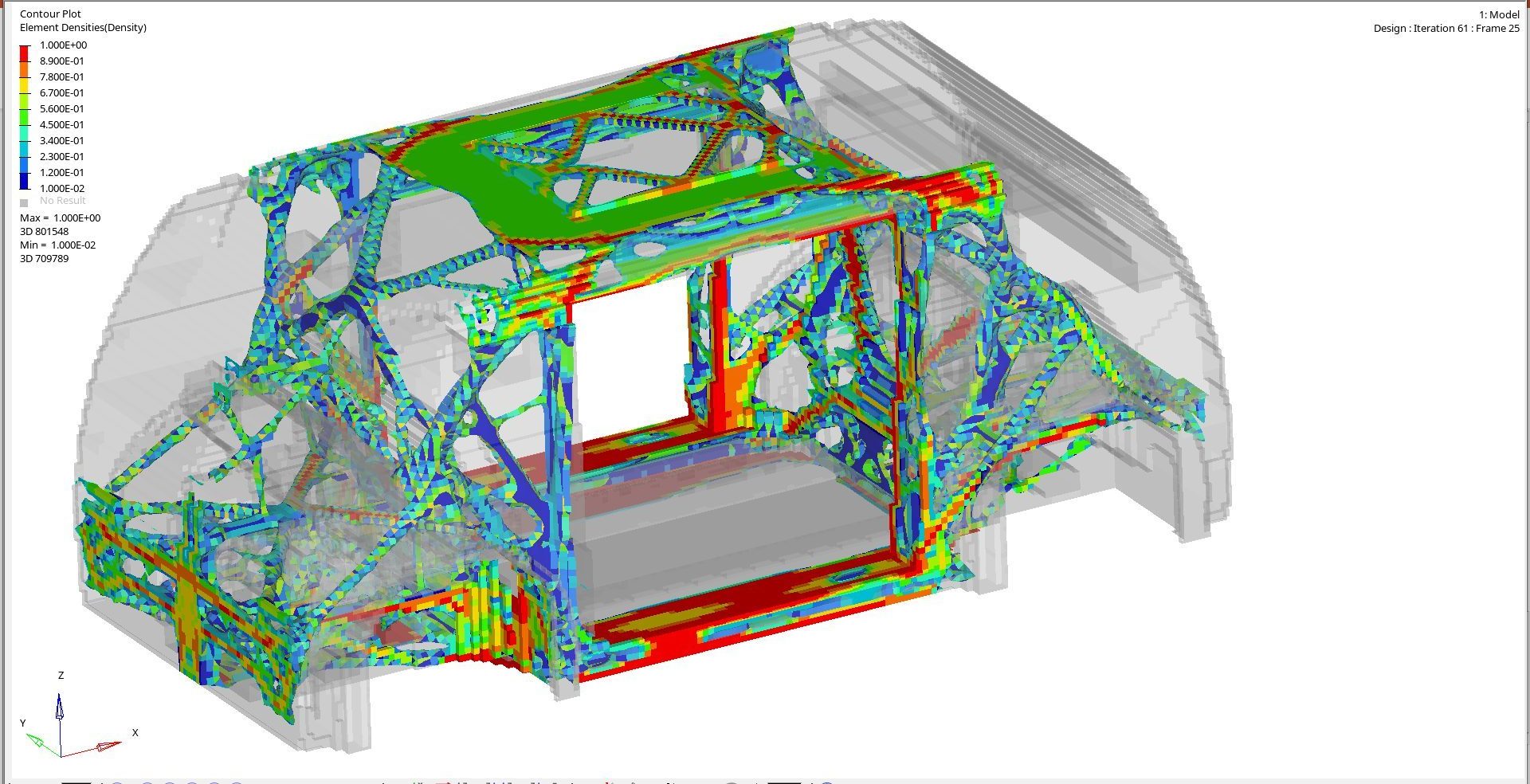
Figure 5: Topology Load Path Optimization. (© WorldAutoSteel 2022)
The approach for achieving body stiffness was as follows. Results are shown in Figure 6 following.
- Topology load path optimization
- Appropriate section size, profiles, part integration and flange / joint design
- Strut towers integrated with key body members, such as A-pillars, vertical dash brace
- Contribution from structural battery frame and battery cover closing, roof structure trusses
- Rigidly connected front and rear subframes
- Optimized joining and use of structural adhesives
- Capitalizing on the Inherent high modulus of steel
Figure 6: SEM Torsional Rigidity animation. (© WorldAutoSteel 2022)
Static torsional stiffness 38,000Nm/deg
Global trimmed BIW modes >28Hz
Local attachment static stiffness ten times bushing stiffness
Front Crash Structure Engineered to Balance the Requirements of 56kph USNCAP FFB, IIHS ODB, IIHS SORB and EuroNCAP MPDB Load cases
One of the most challenging aspects of the Steel E-Motive program has been achieving the front crash performance that minimizes occupant injury. The challenge has been compounded by the overall compact size of the vehicle and the short front overhang dimensions, meaning less space to manage and balance the required crush energy with intrusion resistance.
For the IIHS 25% Small Overlap test, we worked from the outset to achieve a barrier “glance off.” The goal is to deflect the vehicle off the barrier by the time the barrier reaches the hinge pillar. This results in a reduced amount of vehicle kinetic energy converted to crush energy. The vehicle continues after the impact with some onward velocity and kinetic energy. This strategy results in reduced intrusion to the passenger compartment and a much lower vehicle pulse (below 20g), which translates into lower occupant injury. We are very excited by this outcome, as in our benchmarking we have not seen many (if any) vehicles of this size managing to achieve a glance off for this test. Figures 7 and the bullets following provide a look at the results.
Figure 7: IIHS 25% Small Overlap test. (© WorldAutoSteel 2022)
- IIHS “good” rating achieved (based on predicted intrusions).
- Our strategy for IIHS Small Overlap test was to achieve a “glance off” the barrier, which is a significant challenge given the vehicle’s short front overhang.
- Front suspension engineered to detach on impact. This is important for achieving glance off.
- Glance off results in some continued onward vehicle velocity after the impact.
- This results in reduced crush energy, lower vehicle pulse and intrusions = enhanced occupant protection
Figure 8 points out features of the front crash structure. Most of the crush energy in FFB and ODB is absorbed by conventional longitudinal mid-rails, which are made of cold stamped, tailor welded blank Dual Phase steels. The plan view angle of the longitudinals has been optimized to provide load reaction early in the SORB event while remaining largely inside of the SORB barrier.
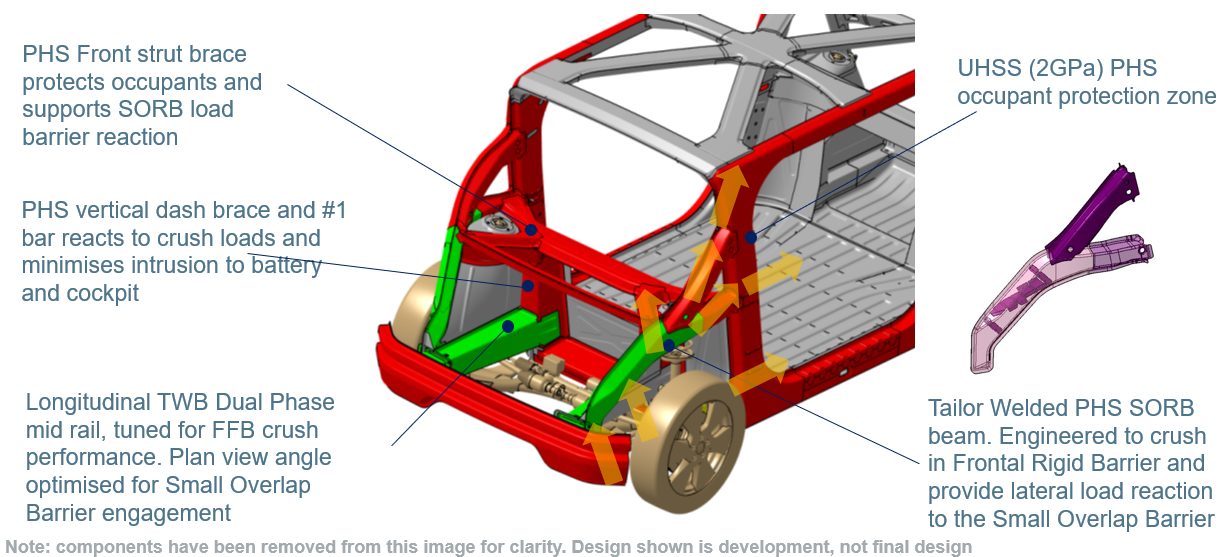
Figure 8: Front crash structure engineered to balance the requirements of 56kph USNCAP FFB, IIHS ODB, IIHS SORB and EuroNCAP MPDB load cases. (© WorldAutoSteel 2022)
Following in Figures 9 and 10 are animations of the FFB results:
|
Figure 9: USNCAP 56kph Rigid Barrier – Top View. (© WorldAutoSteel 2022) |
|
Figure 10: USNCAP 56kph Rigid Barrier – Side View. (© WorldAutoSteel 2022) |
MaaS vehicles will need to accommodate quick ingress and egress as well as provide comfort and safety for the occupants. Consequently, we have flipped the front occupant around to a rear facing configuration and provided a B-Pillarless wide door aperture to enable comfortable and quick access for passengers. This changes the approach required for occupant protection in a front crash. Effectively we are dealing with a high-speed rear impact situation for the occupant. Current rear impact tests cover lower speed rear end shunts. Figure 11 notes the key points and challenges that Steel E-Motive is designed to meet.
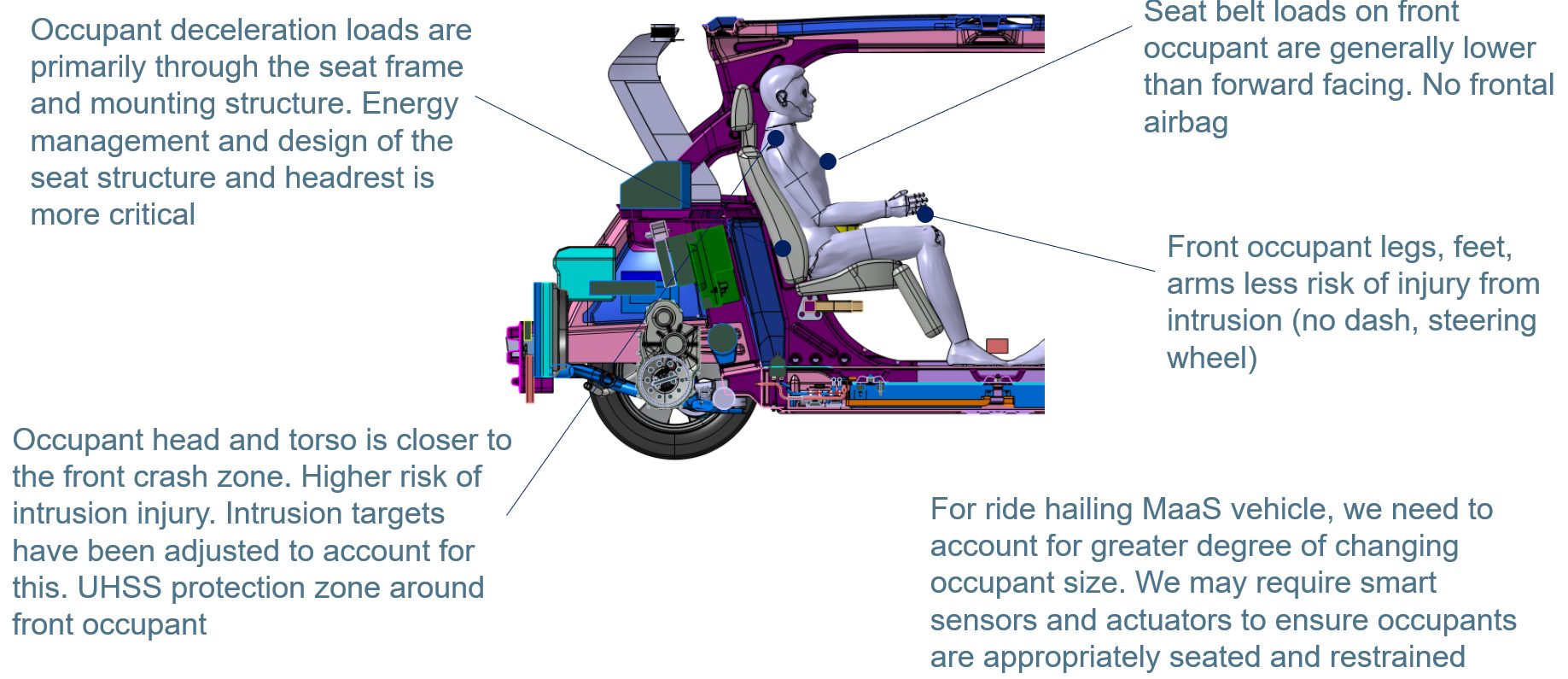
Figure 11: Different approach and considerations are required for the protection of rear facing front occupants. We are effectively addressing a high-speed rear impact event. (© WorldAutoSteel 2022)
Side Crash Structure Consists of Absorption and Intrusion Prevention Zones, Compensating for Large Body Aperture
The side structure includes roll-stamped martensitic door waist rail beams and a one-piece Tailor Welded Blank, Press Hardened Steel door ring outer. A- and C-pillars in line with occupants provide good side impact protection. (You can learn more about the door design in our May blog).
In the section AA schematic in Figure 12 the TRIP690 hydroformed tube interlocking door B-pillar is shown (wrapped over the rocker and cantrail). The load travels through the side impact crush “hex” beam, which is a two-piece roll formed DP590 component.
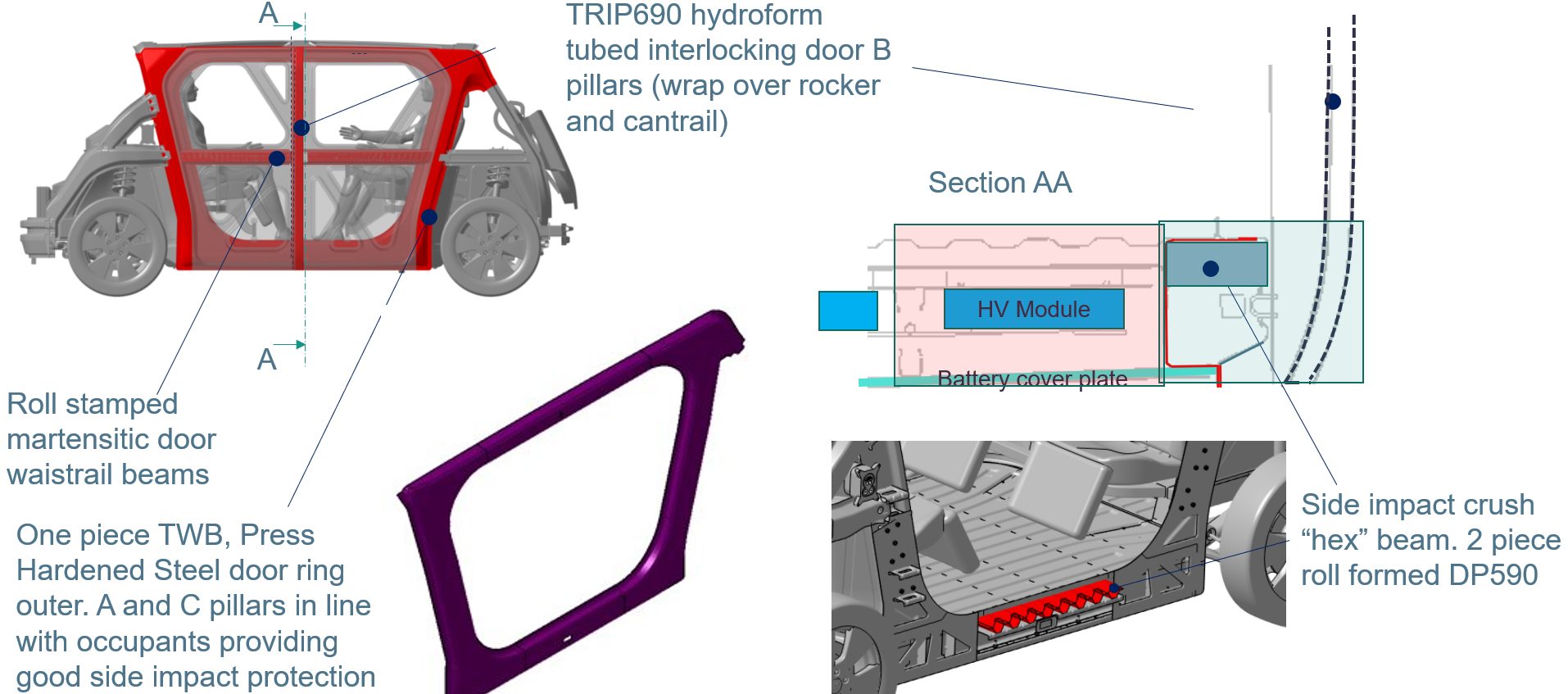
Figure 12: Side crash structure consists of absorption and intrusion prevention zones, compensating for large body aperture. (© WorldAutoSteel 2022)
Steel E-Motive Design Demonstrates Good Side Crashworthiness and Good Levels of Occupant and Battery Protection
In addition to occupant protection tests, additional side impact load cases have been simulated to ensure optimal battery protection. The design maintains a less than 30 mm clearance to the battery.
In reviewing the design according to IIHS standards and based on the predicted intrusions, we are confident this vehicle would achieve an IIHS “good” rating. See Figures 13 and 14 following:
Figure 13: USNCAP 32kph side pole (battery protection). (© WorldAutoSteel 2022)
In addition to occupant protection test, additional side pole load cases to ensure battery protection
>30mm clearance to battery maintained
Figure 14: IIHS 60kph side barrier II (occupant protection). (© WorldAutoSteel 2022)
IIHS “good” rating (based on predicted intrusions).
Total Cost of Ownership: Vehicle and Body Is Designed for Conventional Fabrication and Assembly Processes
The Steel E-Motive body has been designed with low cost in mind to provide the foundation for a lower total cost of ownership for fleet owners. The steel body design is optimized to maximize material utilization and minimize scrap rate. Steel E-Motive is suitable for >250,000 units/year production and is compatible with existing global automotive manufacturing facilities using conventional press and fabrication tools. We are also using Life Cycle Assessment as an integral part of the engineering process to ensure that Steel E-Motive is responsible for the lowest possible emissions throughout its entire life cycle. We will report on environmental performance and sustainability as a part of our final results.
Steel E-Motive Key Outcomes
The Steel E-Motive program is delivering an exciting futuristic vehicle, optimized from the ground up for autonomous MaaS application. We are addressing key challenges through careful design, application of simulation tools and efficient use of the latest Advanced High-Strength Steels and fabrication processes. Steel’s inherent characteristics of low production emissions, lightweighting capabilities for mass efficiency, infinite recyclability and product durability underscores its suitability as an integral part of stakeholder strategies to offer sustainable mobility solutions, today and in the future.
Be sure to follow us on our journey as we enter our final months of design, engineering and reporting by subscribing at the Steel E-Motive website. We welcome your questions about this program using the Comment box below.
Images are not for use without permission. Contact steel@worldautosteel.org.
homepage-featured-top, main-blog, News
There is interest in the sheet metal industry on how to adopt Industry 4.0 into their legacy forming practices to significantly improve productivity and product quality. Figure 1 illustrates four important variables influencing part quality: material properties, die friction response, elastic deflection of the tool, and press dynamic characteristics. These variables are usually difficult to measure or track during the production runs. When these variables significantly influence the part quality and the scrap rate increases, the operators manually adjust the forming press parameters (speed and pressure), lubricant amount, and tooling setup. However, these manual adjustments are not always possible or effective and can be costly for the increased part complexity.

Figure 1: Important variables influencing the stamping quality.H-35
The ultimate vision for Industry 4.0 in sheet metal forming is an autonomous forming process with maximum process efficiency and minimum scrap rate. This is very similar to the full self-driving (FSD) vision of the electric vehicle today. This will be valuable for the automotive industry that has to process large production volumes with various steel grades.
For example, normal variations of the incoming material properties for Advanced High-Strength Steel (AHSS) may have a significant effect on part quality associated with necking, wrinkling, and cracking, which in turn drastically increases the production cost. This variation of the incoming material properties increases uncertainty in sheet metal forming by making consistent quality more challenging to achieve, thereby increasing the overall manufacturing cost. A nondestructive evaluation (NDE) can be a useful tool to measure incoming material properties.
There are several types of NDE sensors. Most of the sensors need further development or are not suitable for production applications. However, some of the NDE sensors, such as the eddy current tools, laser triangulation sensors equipment, and equipment developed by Fraunhofer IZFP called 3MA (micromagnetic, multiparametric microstructure, and stress analysis), have already been applied to a few limited production applications. These sensors can be used to provide data during production to select the optimal parameters. They also can be used to obtain material properties for finite element model (FEM) analysis. Studies in deep drawing of a kitchen sink production used a laser triangulation sensor to measure the sheet thickness and an eddy-current sensor to measure the yield strength, tensile strength, uniform elongation, elongation to break, and grain size of the incoming material. The material data is used as an input for simulations to generate the metamodels to determine the process window, and it is used as an input for the feed-forward control during the process.K-27
Figure 2 shows how NDE tools are used for feed-forward controls and cameras for feedback control to determine the optimum press setting on sink forming production.

Figure 2: Process control for sheet metal forming of kitchen sink production.H-36
Another study proposed the use of Fraunhofer’s 3MA equipment to determine the mechanical properties of incoming blanks for a sheet forming process. The 3MA sensor correlates the magnetic properties of the material with the mechanical properties and calibrates the system with the procedure outlined in Figure 3. The study showed a good correlation between the measurements from the sensor and the tensile testing results; however, the sensor should be calibrated for each material. Also, the study proposed to use a machine-learning algorithm instead of a feed-forward control to predict the most effective parameters during the drawing process.K-28

Figure 3: Calibration procedure for 3MA sensors.K-28
Technologies associated with Industry 4.0 have a natural fit with AHSS. The advanced slide motion capabilities of servo presses combined with active binder force control can be paired with stamping tonnage and edge location measurements from every hit to create closed-loop feedback control. With press hardening steels (PHS), vision sensors and thermal cameras can be used for controlling the press machine and transfer system.
Have a look at the Dr. Kim’s detailed article on Industry 4.0 for more examples of NDE sensors, as well as information on applying Industry 4.0 to forming process controls.