Blog, homepage-featured-top, main-blog, News
The transportation industry’s contribution to greenhouse gas (GHG) emissions and global warming is well documented and understood. Vehicle OEMs, fleet operators, and transport users all have responsibilities to reduce environmental impacts on the planet and contribute to meeting global emissions regulations.
Mobility as a Service (MaaS) solutions like WorldAutoSteel’s flaghip Steel E-Motive (SEM) program have the potential to contribute to a reduction in GHG emissions, helping to achieve these global targets and specific policy objectives. The Steel E-Motive engineering report, released in 2023, addresses the impact of emissions reduction using Life Cycle Assessment, with key results summarized in this article.
Introduction to Life Cycle Assessment
Life Cycle Assessment (LCA) is a methodology that evaluates the environmental impact of a product across its entire lifecycle. By understanding the impact across the entire vehicle life cycle, vehicle manufacturers evaluate trade-offs and assess the net impact of the product they’re using.
Cradle-to-grave assessments utilize a boundary that includes impacts from the production phase (including raw material extraction and vehicle production), the use phase (including fuel or electricity as well as consumables like tires and fluids) and the end-of-life phase, which could include disposal and/or recyling of the product, as shown in Figure 1. We applied LCA throughout the development of the SEM concept.
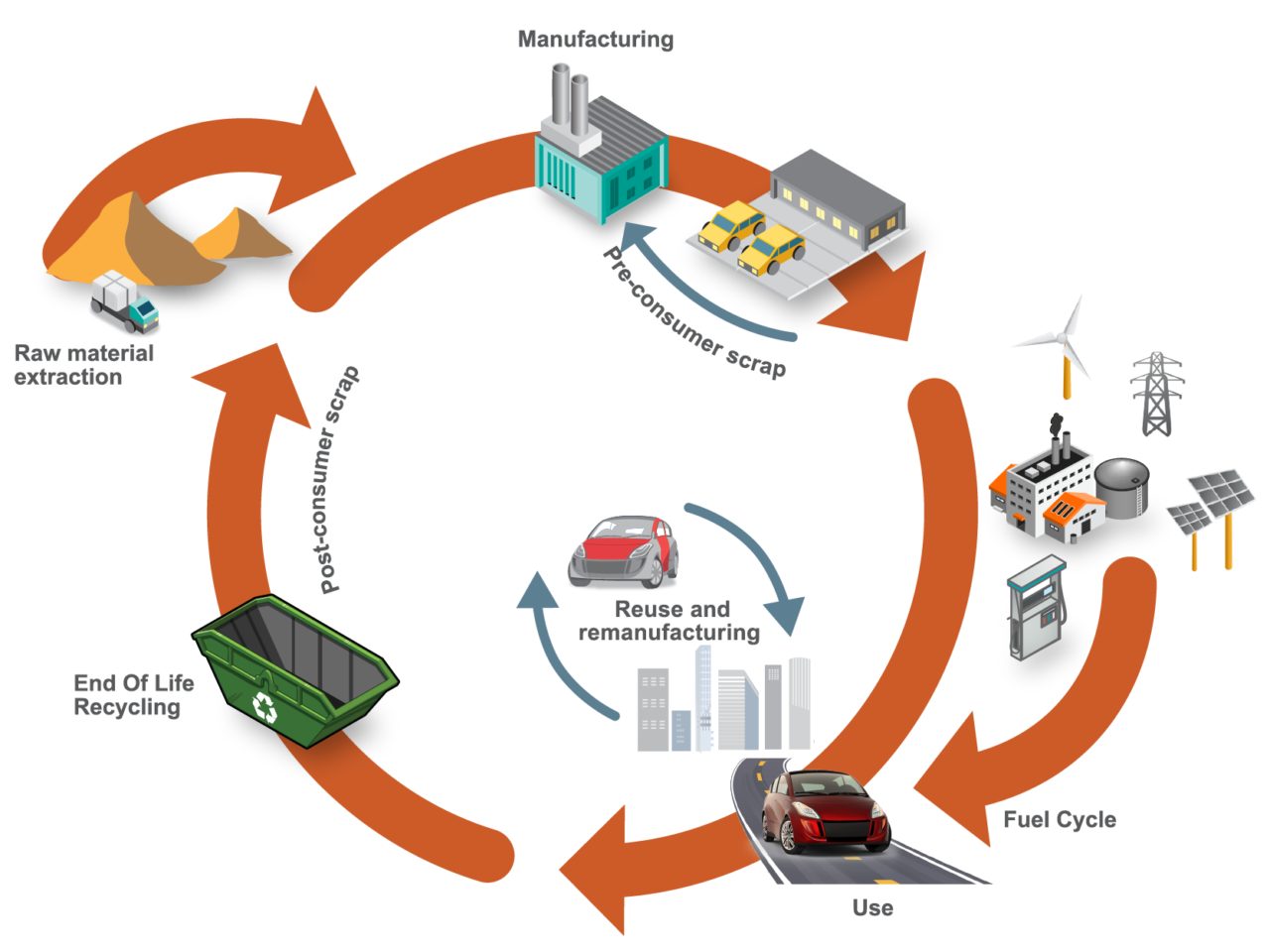
Figure 1. SEQ Figure \* ARABIC 1 Life Cycle Assessment, considering the entire life of the vehicle, from raw material extraction to end of life
LCA can cover a range of environmental impacts; however, for the SEM program, we focused on GHG emissions through the GWP-100 indicator and total energy consumption using Cumulative/Primary Energy Demand and Fossil Energy Consumption indicators.
Reference Taxi (Baseline) Vehicle
A key consideration in LCA calculations is establishing an appropriate reference vehicle. For this program, the following criteria was used:
- Present day (~2020) battery electric vehicle (BEV) operating in taxi mode with a driver and one occupant with vehicle/battery lifetime assumptions of 300,000km, and use of 100 percent conventional steel/aluminum.
- Vehicle end-of-life methodology using the Avoided Burden Approach, where recycled metals are assumed to displace equivalent quantities of their virgin counterparts and assigned corresponding emission and energy demand credits.
- Assumption of 50 percent pyrometallurgical recycling for the battery packs.
- Estimated reference taxi vehicle curb weight using the statistical reference data study (Figure 2), resulting in an estimated curb weight of 1,949kg.
- Material utilization based on data from a similar vehicle specification, as shown in Figure 3.
- Vehicle occupancy rate assumptions of 1.4, based on a combination of both “empty” and passenger-carrying journeys.
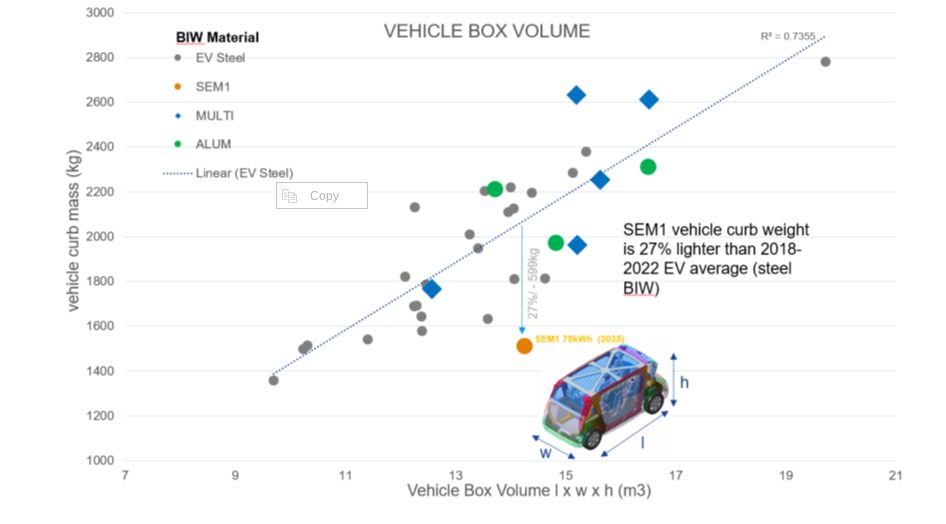
Figure 2. Vehicle curb weight versus box volume comparison. Reference vehicle data; source www.a2mac1.com
Steel E-Motive “Default” Vehicle
SEM vehicle life cycle calculations assume a hypothetical 2030 manufacture and start-of-operation date of 2030 to 2035. We updated the electricity grid supply mix to include the average of the International Energy Agency (IEA) scenario estimates for 2030 and 2040.
- We applied the nominal SEM1 vehicle curb weight of 1,512kg in the LCA model, and updated the vehicle Bill of Materials.
- As with the reference vehicle, we adopted the Avoided Burden Approach as the default for end-of-life calculation.
Life Cycle Assessment Results
Figure 3 below highlights absolute calculated life cycle GHG emissions, in units of kgCO2e/ passengerꞏkilometer studied, with the individual contributions of vehicle manufacturing, vehicle use, and end-of-life phase presented.
The analysis evaluated two reference/baseline conditions and nine SEM sensitivity studies, see Figure 4. These included alternative assumptions on LCA end-of-life modeling methodology, lifetime vehicle activity (and battery lifetime), alternative operational energy consumption sensitivities, sensitivities on the use of ‘green’ steel, and vehicle occupancy rates.
The accompanying pie chart shows the breakdown and contributions to the vehicle manufacture GHG for the baseline SEM scenario (2).
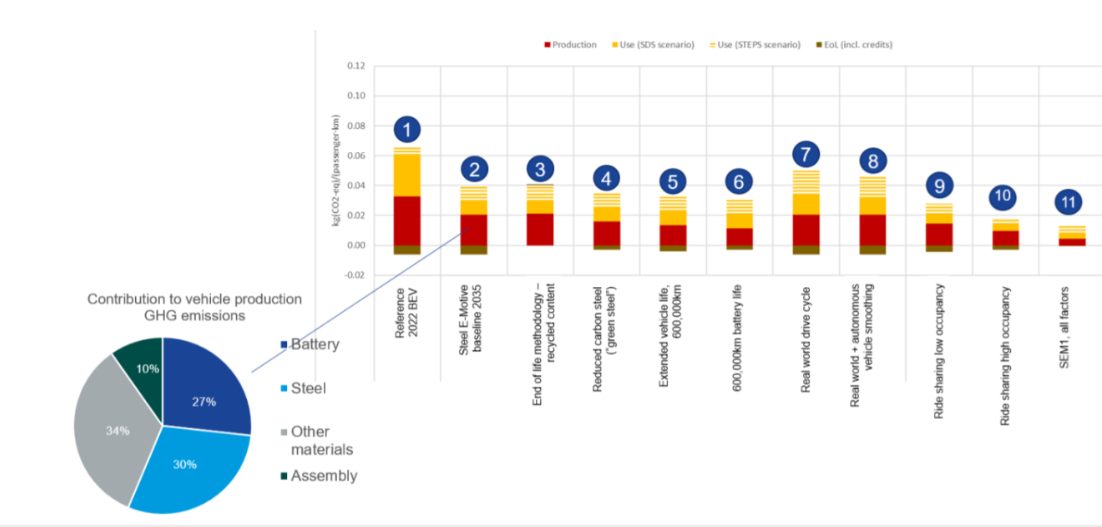
Figure 3. SEQ Figure \* ARABIC 2 life cycle assessment GHG results
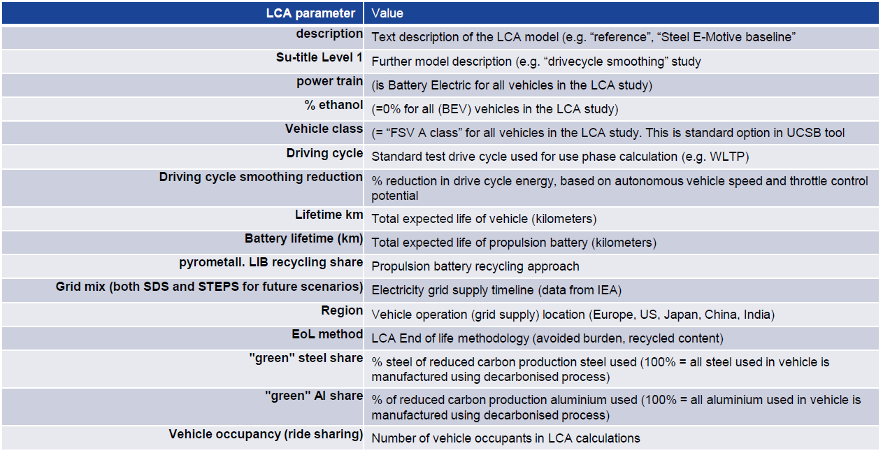
Figure 4. Reference/baseline conditions and SEM sensitivity studies
Life Cycle Assessment Conclusions
Based on the parameters outlined, applying LCA to SEM concept demonstrated the designs’ potential to reduce lifecycle greenhouse gas emissions by up to 86 percent compared to a present-day battery electric vehicle operating as a taxi.
This potential can be realized by adopting the following measures:
- Reducing vehicle production and manufacturing embedded emissions by utilizing 100 percent reduced carbon (“green”) steel
- Improving battery technology and increasing the use of renewable electricity in battery manufacturing; as well as increasing/improving battery recycling
- Ensuring the vehicle weight of autonomous vehicles is managed, and the potential weight reduction benefits realized and implemented. The SEM body structure and battery housing demonstrate good weight efficiency.
- Increasing the overall lifespan of the vehicle and battery. The fatigue and durability properties of AHSS can enable enhanced vehicle lifetime. The SEM battery design allows easy replacement of specific modules, enabling an overall extended battery life.
- Autonomous vehicle control smooths the driving cycle. The vehicle acceleration and deceleration rates can be optimized to match the driving conditions and road topography, reducing energy consumption and subsequent GHG emissions.
- Increasing passenger occupancy rates to at least three per vehicle via MaaS.
The projected net GHG emissions for the SEM vehicle operating with the flexibilities described above already represent a significant reduction when compared to the current baseline.
Achieving net zero emissions would require additional measures like offsetting manufacturing impacts (e.g., through compensatory credits from atmospheric carbon capture and storage) and transitioning to a 100 percent renewable electricity grid.
Moving Toward Net Zero
Taking a Life Cycle Assessment approach to the SEM concept demonstrates the possibilities for engineering future mobility vehicles that continue to move us closer to a net zero future. For more information about the Steel E-Motive program, download the engineering report here: https://bit.ly/SEM_Eng_Report
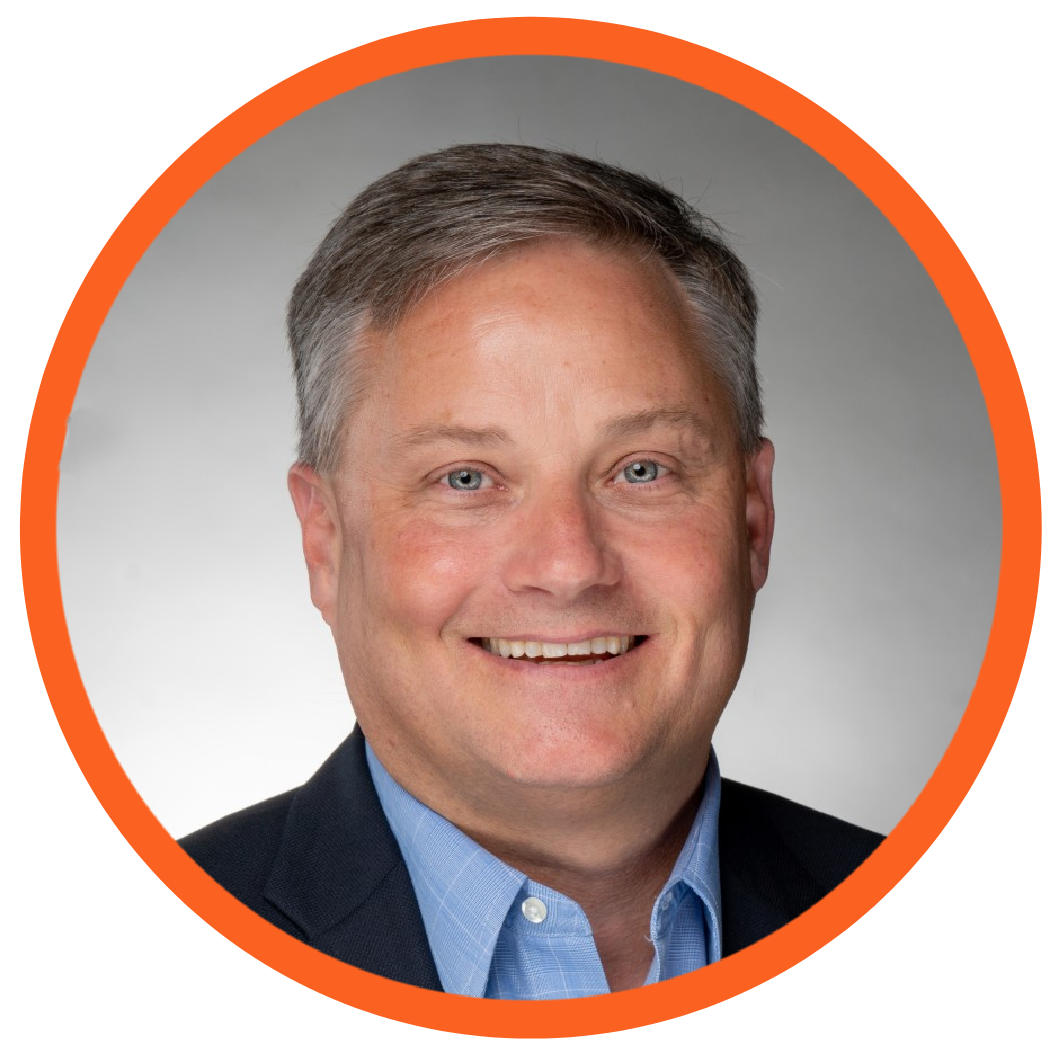
Thanks go to Russ Balzer for his contribution of this article to the AHSS Insights blog. As.technical director at WorldAutoSteel, he leads technical programs and oversees the organization’s work in research, modeling, and advocacy for Life Cycle Assessment in the automotive sector. An LCA Certified Professional through the American Center for Life Cycle Assessment (ACLCA), he also acts as the WorldAutoSteel liaison to the worldsteel LCA Expert Group.
AHSS, Blog, main-blog, News
Net Zero Emissions by 2050 – it’s a goal for future mobility that can seem distant and daunting. But over the past five years, WorldAutoSteel’s global automotive steel suppliers have conducted extensive research that illuminates a path forward. The Steel E-Motive concept – borne of this research – can be a catalyst for reaching the Net Zero goal.
Urbanization and changing attitudes towards vehicle ownership point to new transport opportunities in megacities worldwide. Mobility as a Service (MaaS) – characterized by autonomous, ride-sharing-friendly EVs – can be the comfortable, economical, and sustainable transportation solution of choice thanks to the benefits that modern steels offer, which will foster the higher vehicle occupancy that is critical to Net Zero ambitions.
Here, we break down the many benefits of the Steel E-Motive vehicle.
The Key Steel E-Motive Vehicle Features for Future Mobility
The Steel E-Motive Vehicle features seven key Advanced High-Strength Steel structural innovations to create a safe, economical vehicle.
- A B-Pillarless open-body structure offers excellent comfort, accessibility and easy ingress/egress.
- The Short Front Crash Zone design meets all global high-speed frontal crash requirements.
- The AHSS Extended Front Passenger Protection Zone provides excellent cabin intrusion protection for occupants.
- The Small Offset Crash Glance Beam minimizes the energy pulse into the occupant cabin, reducing the potential for passenger injuries.
- Hex beam energy absorbers provide superior battery protection for both side pole and deformable barrier crashes.
- The Scissor Door with Virtual B-Pillars offers excellent passenger visibility while saving mass and costs.
- The Coverless Battery Carrier Frame concept rewards 37% mass savings over benchmarks and 27% cost reduction; it also affords enhanced battery protection from road debris and other floor impacts.
The Steel E-Motive vehicle is created to meet Level 5 autonomy, meaning it is void of driver interfaces and does not require any human attention. With all of these features and more, the SEM architecture affords a spacious, safe, and comfortable cabin for occupants.
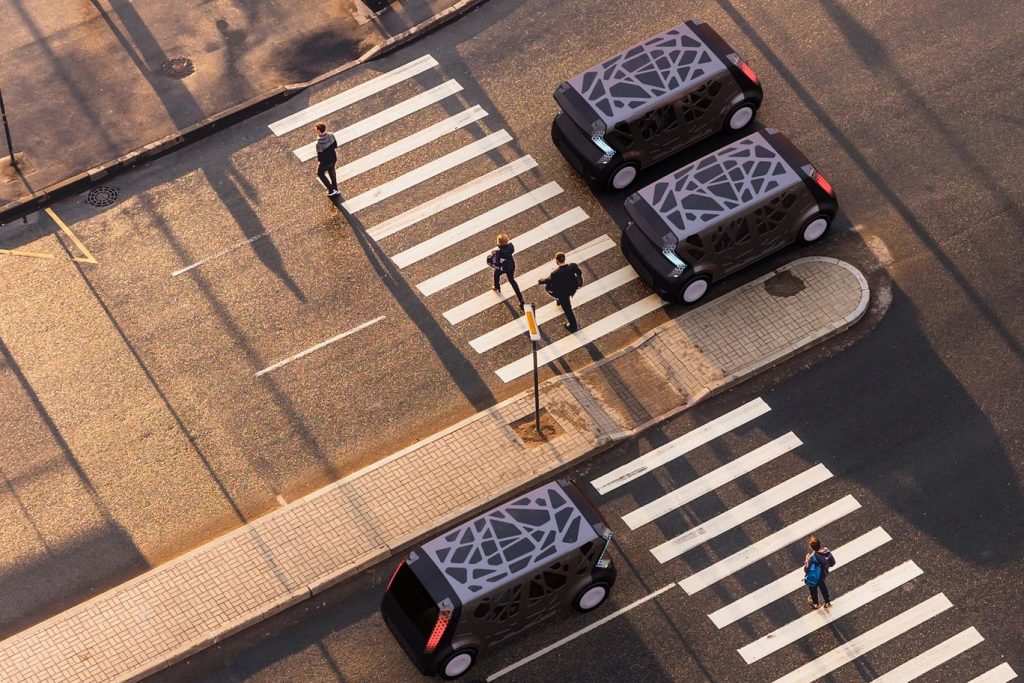
Steel E-Motive concepts are designed to help pave the way to a Net Zero future.
Exceeds Crash Guidelines
The Steel E-Motive vehicle is one of the world’s first autonomous vehicle concepts to validate and report excellent performance measured against the most stringent global crash requirements, which aligns with an IIHS “Good” rating. Modern Advanced High-Strength Steel product and fabrication process innovations enable the vehicle design to exceed these stringent crashworthiness standards while minimizing overall mass and production emissions.
Created to Be Affordable
Considering both production and life cycle costs, Steel E-Motive concepts have low maintenance requirements and are designed to be manufacturable using the world’s global manufacturing infrastructure at costs that support profitable margins, both for the vehicle manufacturer and the mobility service providers. Steel E-Motive is a fully engineered vehicle program that start-up companies can use to significantly reduce their cost and time to market.
Designed with Sustainability in Mind
The viability of any MaaS disrupter is contingent on cost competitiveness versus existing solutions, such as private ownership or taxis.
Moreover, our designs minimize steel thicknesses for lower mass while maximizing material utilization for lower steel production and emissions. Overall, the vehicle design offers the potential for ~86% CO2 emissions reduction when all factors contributing to sustainability are optimized. Autonomy further reduces operating emissions due to drive cycle smoothing.
To achieve our Net Zero future, high-occupancy vehicle usage is crucial and must be appealing for riders and profitable for providers.
Steel E-Motive concepts play a vital role in enabling Future Mobility Solutions THAT ONLY STEEL CAN MAKE REAL. Learn more about the program: https://steelemotive.world/
Blog, main-blog
Here at WorldAutoSteel, we have been studying the changes in the automotive industry for several years, focusing particularly on ride sharing, autonomous, electric vehicles and steel’s role in that marketplace. George Coates, Technical Director for WorldAutoSteel and The Phoenix Group, has been leading that effort and today contributes an article on the disruption of future mobility to the industry and the great opportunities we see for steel in meeting the challenges providers will face. We hope you enjoy the read, and we welcome your thoughts and comments. What changes and impacts do you envision for vehicle manufacture? How do you feel about the world of autonomy?
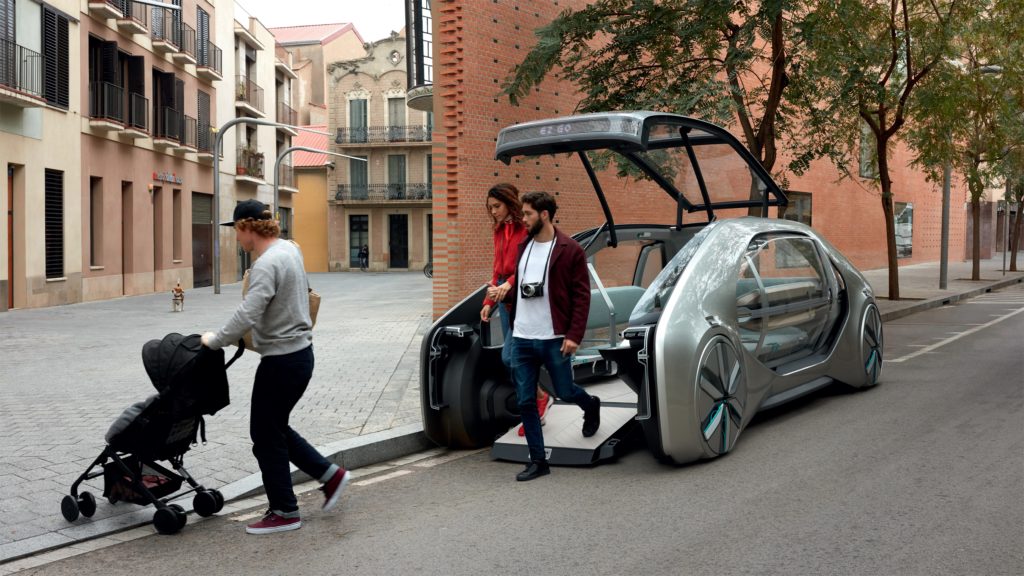
Renault’s Future Mobility concept, the E-Z Go
We’re approaching a critical milestone in automotive history when what we know as normal is about to change significantly. Future Mobility describes the revolution that’s already begun. We’re rethinking transportation from the movement of a vehicle to a more efficient concept for moving people and things. We’re about to discover the social advantages of connected, autonomous, shared and electric vehicles. And we’re completely changing the way we view transportation.
By 2030, electric vehicles (EVs) will be mainstream—not just within the premium segment, as they are today. EV’s will be popular and available across all vehicle variants and prolific in the commercial vehicle industry and in public transportation. Owners and fleet providers will experience the lower costs of electricity, lower maintenance costs, and the lower overall total cost of ownership (TCO). Fully autonomous or self-driving vehicles will introduce design freedom never experienced before, with the removal of the steering wheel, foot pedals and conventional dashboard. Communication and comfort will be re-imagined, with a vehicle that’s no longer designed around the driver but designed to serve the needs and comfort of the occupants, who are now users instead of owners.
With the rise of mobility services such as Uber, Didi, and a host of others, vehicle ownership is fast becoming an option. In a very short time, especially in urban areas such as China’s mega-cities, it is becoming cost-efficient to subscribe to a monthly ride share service for all of your transportation needs.
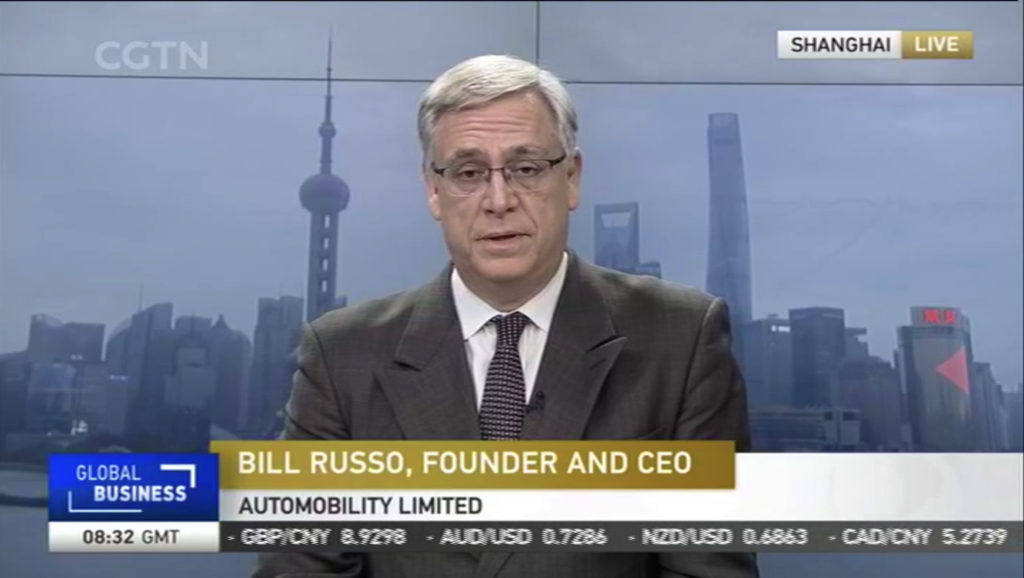
Bill Russo, CEO, Automobility LTD
Bill Russo, CEO of China-based Automobility, in a December 2018 article, Competing in the Digital Internet of Mobility, notes that the digital connectivity of these vehicles will open up profit opportunities well beyond the vehicle hardware. He says “An expanded understanding of mobility use cases and tailoring of the mobility hardware ‘form factor’ to the particular mobility need will be a way to create a value proposition that is rooted in the unique riding experience. In the user-centric world where users are passengers, the focus shifts from traditional driver-centric design to a user-centric productivity space. Instead of traveling in the cockpit, we will move in business class or economy class, depending on our preferences and budget.” Cities will be re-imagined in new social opportunities associated with autonomy, as these vehicles will serve the under-served, and infrastructures will shift in purpose to move people, as opposed to moving vehicles.
Where does steel fit?
The steel industry plans to be right in the middle of this revolutionary change. Fleet owners who provide ride hailing and ride sharing services need to manage the total cost of ownership, while maximizing the user experience for added revenue. To be profitable, they’ll want durable, lasting structures that are affordable to own, provide the user motion as well as emotional comfort, while being efficient to operate, and environmentally friendly – and steel is the only material that meets all these requirements.
On Camera Now: George Coates, Technical Director, WorldAutoSteel and Phoenix Group from worldautosteel on Vimeo.
As always, steel is needed for the crash safety structures, and now add battery protection. Our market intelligence shows that due to the high cost to municipalities and regional governments, autonomous-only vehicles will be limited to dedicated areas for a long time to come. Meanwhile, vehicle-to-vehicle and vehicle-to-infrastructure connectivity will result in dramatic improvements in accident avoidance and reduced fatalities.
Because it will take many years before all vehicles on the road have these technologies in play, the need for passive safety will remain for the foreseeable future. Developing a structural design for the passenger compartment becomes challenging, since there’s a now a need to strike a balance between occupant safety and the occupant freedom. This is enabled by removing the driver and controls from the interior. Steel will be needed to provide the unique properties of both crash energy absorption and deflection, while also managing the loads associated with passengers in multiple and diverse seating configurations. Steel has the ability to provide needed strength while keeping the material thin, which lends more room in the passenger cabin for new seating arrangements and more seats. And battery housings made from steel will provide structural integrity for crash management, while also preventing battery pack damage and leakage.
Lightweighting will continue to be important in an effort to balance smaller battery sizes with maximum range. The steel industry has been and will continue to develop products, such as the ever-growing family of Advanced High-Strength Steels (AHSS), to meet both the mass reduction and the safety targets, affordably. With content innovation and the amazing flexibility of the Iron (Fe) element, researchers still have vast development possibilities for new steels that are stronger, more formable and cost effective.