Joining, Projection Welding
As with resistance spot welding in automotive applications, projection welding also is used to join two overlapping sheets of relatively thin metal. The process involves pressing a projection or number of projections in one of the plates and welding the two plates together at the projection locations.
The method can also be used for welding metal sheet to the ends of bars, rods or pipes, or for welding bolts, nuts, and other attachments to sheets. Such attachments are being used widely in the automotive industry. Wire grids (i.e. the crossing points of the wires) are also particularly suitable for projection welding (it is also called cross wire welding).
A modern car body may contains some 300 welded and punched fasteners, such as bolts, nuts, and studs. The quality of the attachment of these fasteners to the stamped body components is critical for the final product’s safety and reliability. Crucial components such as the front and rear axles are mounted to such fasteners, the seat belts and steering column are anchored to them, and they provide grounding for electrical wires.L-25
As noted, projection welding is similar to resistance spot welding. However, in the Resistance Spot Welding process, the size of the contact surface of the electrode cap tip determines the current flow, whereas in projection welding, the current flow is constricted to the embossed or machined projection as shown in Figure 1. Both AC and DC power sources are suitable for fastener welding. The heat balance for projection welding is affected by the following factorsA-11:
- Projection design and location
- Thickness of the sheet to which the fastener is attached
- Thermal and electrical conductivities of the metals being welded
- Heating rate
- Electrode alloy type
As compared to Resistance Spot and Seam Welding, Resistance Projection Welding is capable of welding much thicker parts, as well as parts with a significant thickness mismatch. As a result, it is often considered as a potential replacement for arc welding processes such as GMAW. One of the reasons for this is the drastic reduction in welding time that can be achieved. For example, a typical automotive part that might require several minutes or more of welding with the GMAW process may have the potential to be welded in less than a few seconds with the Resistance Projection Welding process. This is because the entire weld or multiple welds can be made at the same time in a single fixture. Another advantage of the process, relative to spot welding, is that there is less wear and tear on the electrodes.
Different types of projections made by different methods are shown in Figure 2. It is important to note that the types of projections that are extensions of the part are known as solid projections (2-B and 2-D) and can only be produced by a machining or forging process, whereas the other projections are more easily produced by stamping with a punch and die. Projections produced with a punch and die usually involve the formation of a molten nugget during welding but not always. The solid projection designs mostly result in solid‐state welds that occur via a forging action as the projection is heated and pressure applied. A common Projection Welding application that uses solid projections involves the attachment of a wide variety of nuts, bolts, and fasteners. Many fasteners used on automobiles are attached this way.
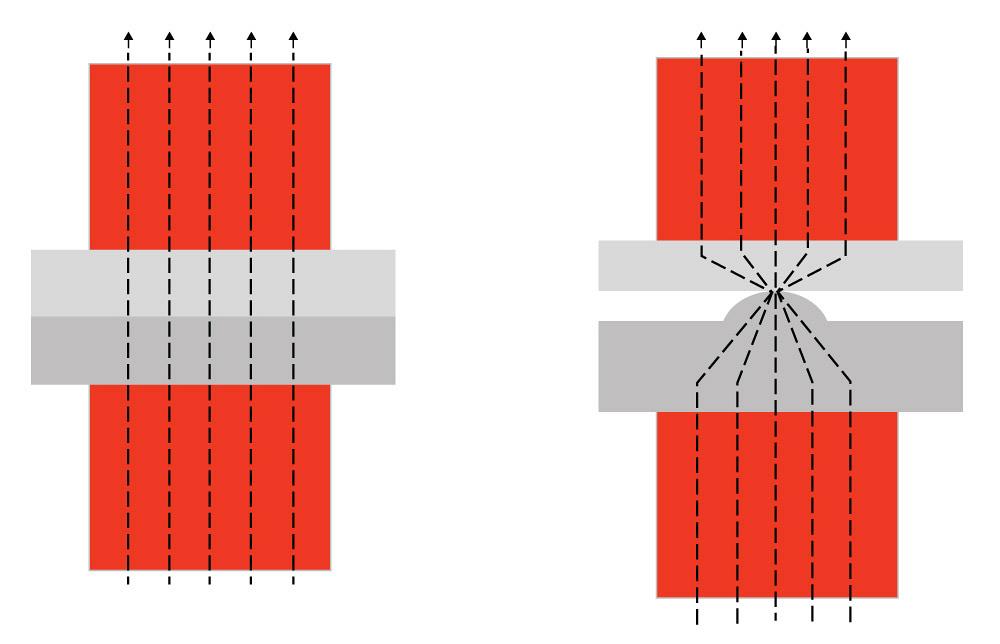
Figure 1: Welding current flow concentration due to projection geometry.
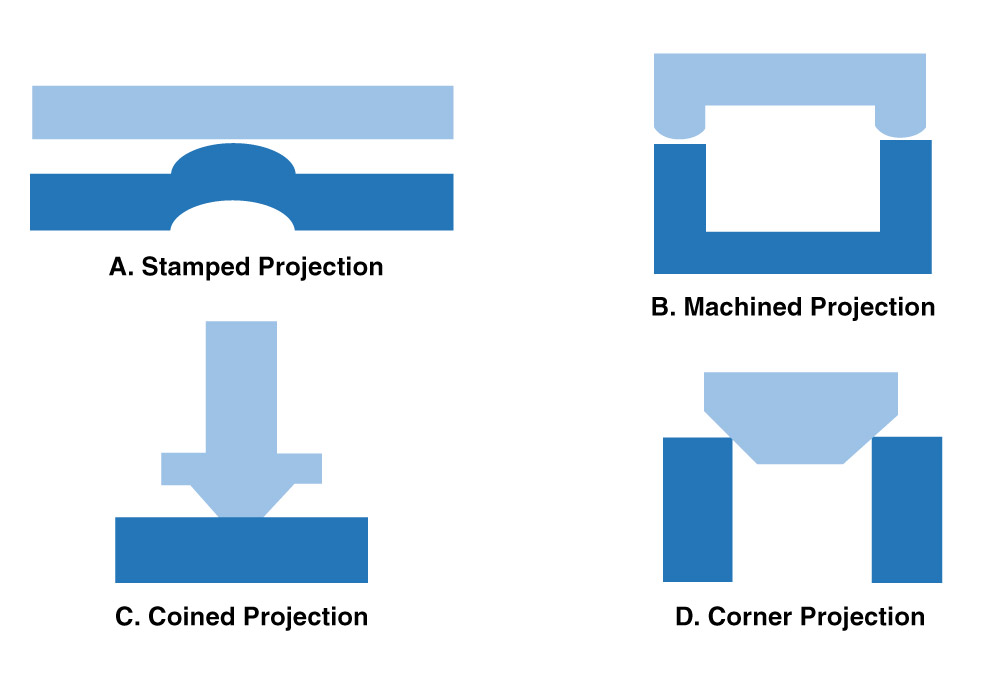
Figure 2: Typical projection types and designs.
Projection Welding
This paper summarizes a paper, entitled “Resistance Welding Projection Methodologies as Applied to Hot-Stamped Boron”, by D. Crist, et al.C-11
The study focuses on two different Resistance Projection Welding approaches and compares the welding parameters, mechanical properties and destructive results. Table 1 lists the secondary impedance measurements and Table 2 lists the material information for the PHS-CR1500T-MB-AS.
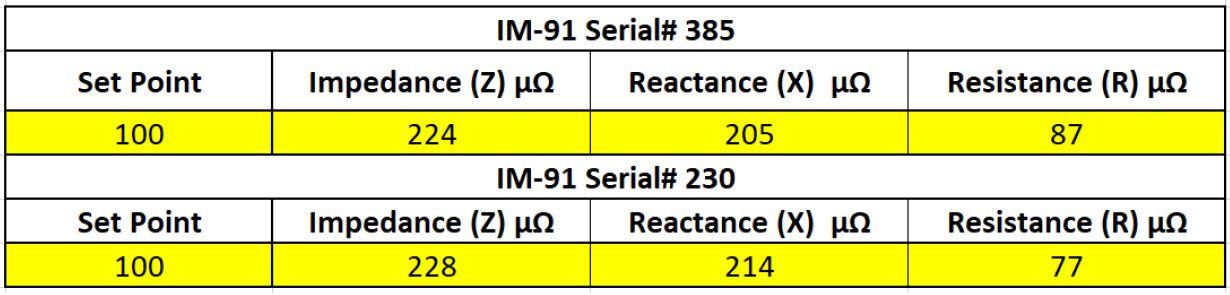
Table 1: Secondary Impedance Measurements.C-11
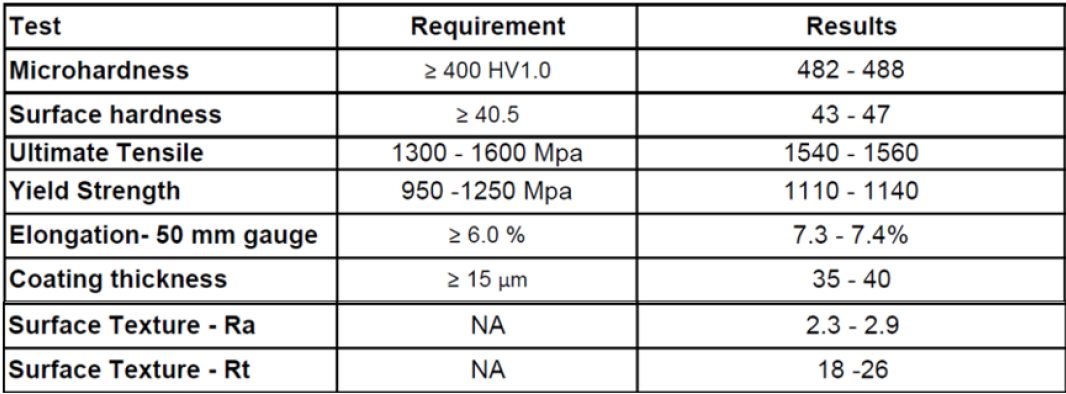
Table 2: Coupon Material Information.C-11
The thickness of the material chosen was 1.80 mm and the weld coupon dimensions are shown in Figure 1.
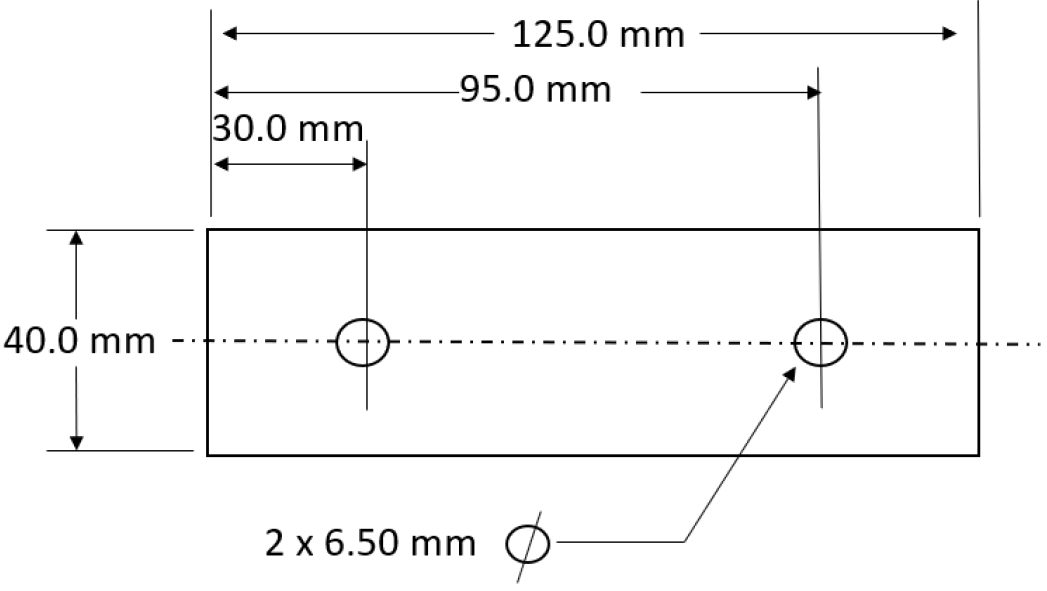
Figure 1: Weld Coupon Dimensions (not to scale).C-11
The projection weld nut used for testing is an M6 hex flange, three projections, non-piloted weld fastener. Detailed dimensions are shown in Figure 2.
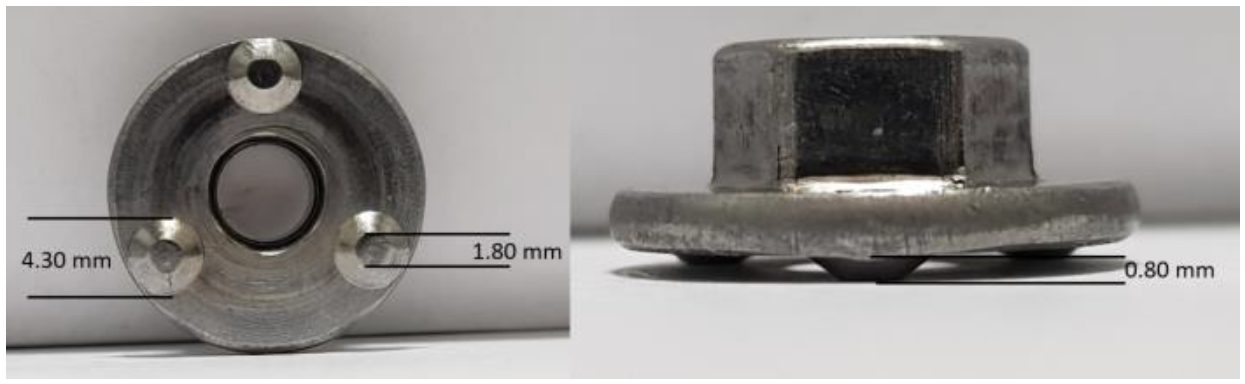
Figure 2: Projection Weld Nut.C-11
Table 3 lists the weld schedule methodologies tested in this study. Table 4 shows the optimized weld schedules based upon push-off strength and visual weld flash level.

Table 3: Weld Schedule Development.C-11

Table 4: Optimized Weld Schedules.C-11
Repeatability testing for both Methods A and B are shown in Figures 3 and 4. The data produced for Method B shows a higher average of repeatability.
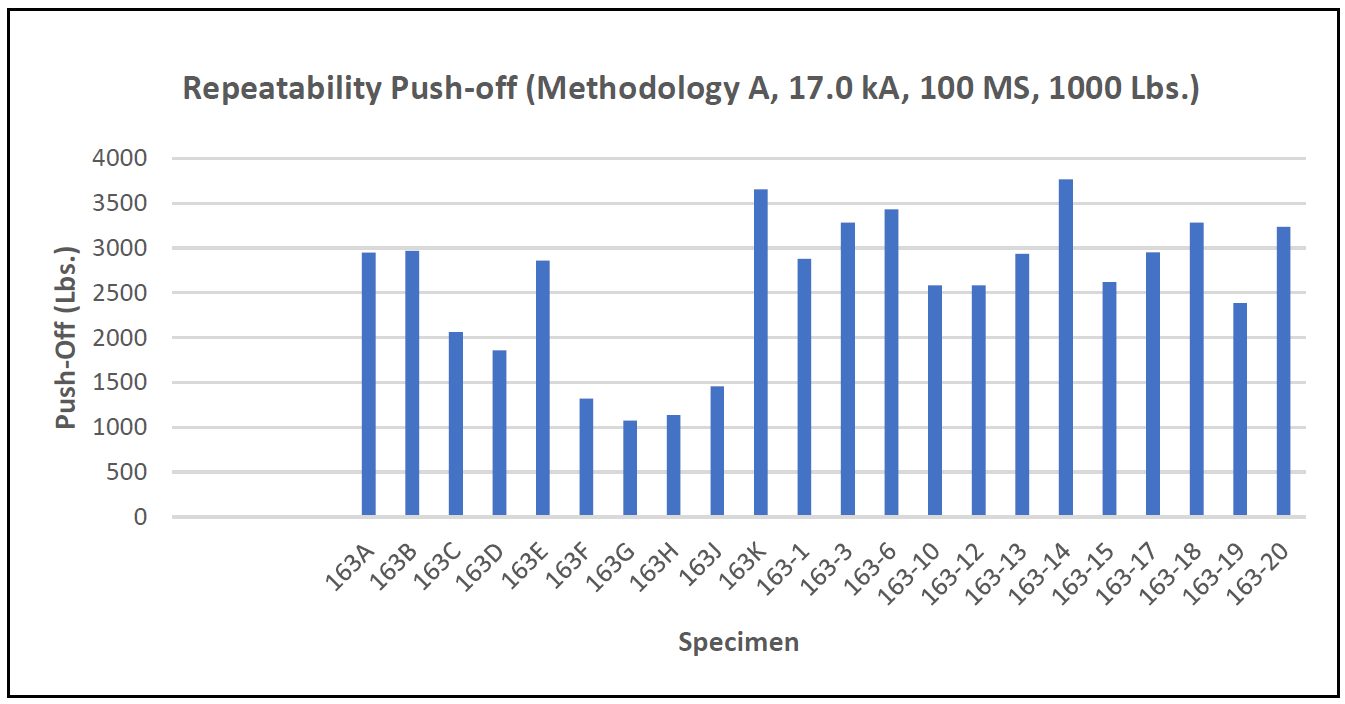
Figure 3: Repeatability Push-Off Method A.C-11
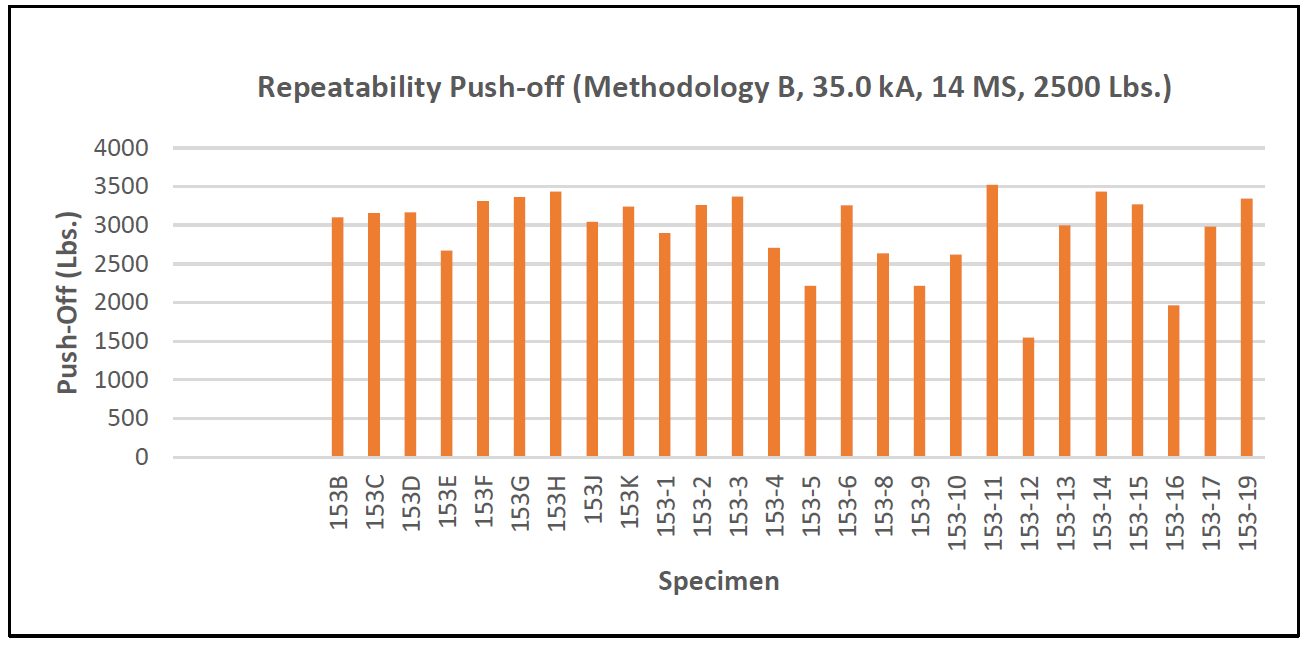
Figure 4: Repeatability Push-Off Method B.C-11
Two different failure methods were observed. Figure 5 shows full thickness buttons were pulled thought the flange for Method A and Figure 6 demonstrates partial thickness failure for Method B.
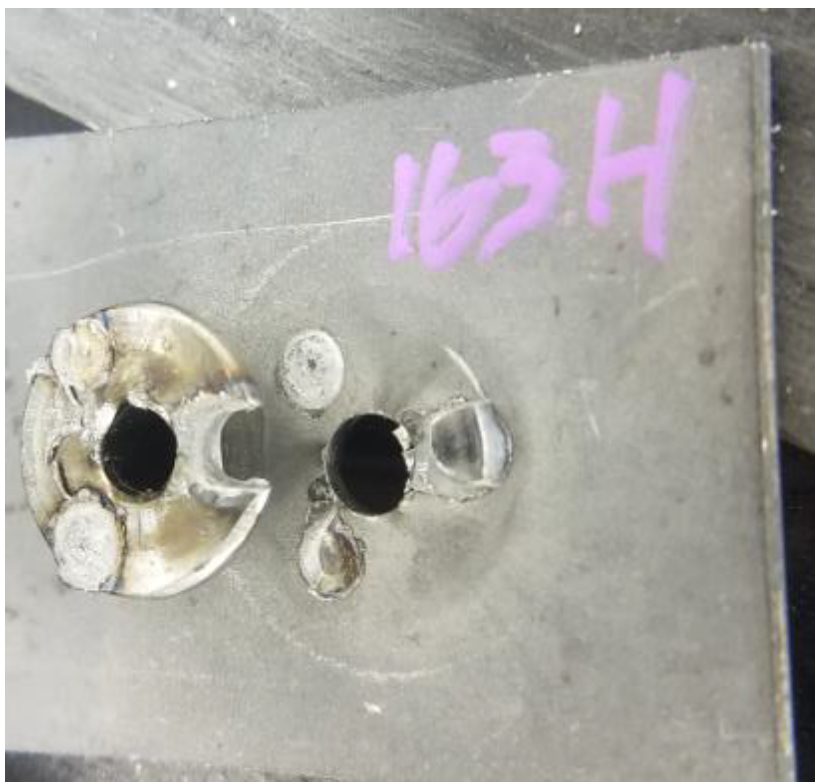
Figure 5: Method A (17.0 kA, 100 ms, 1000 lb.) Common Failure Mode.C-11
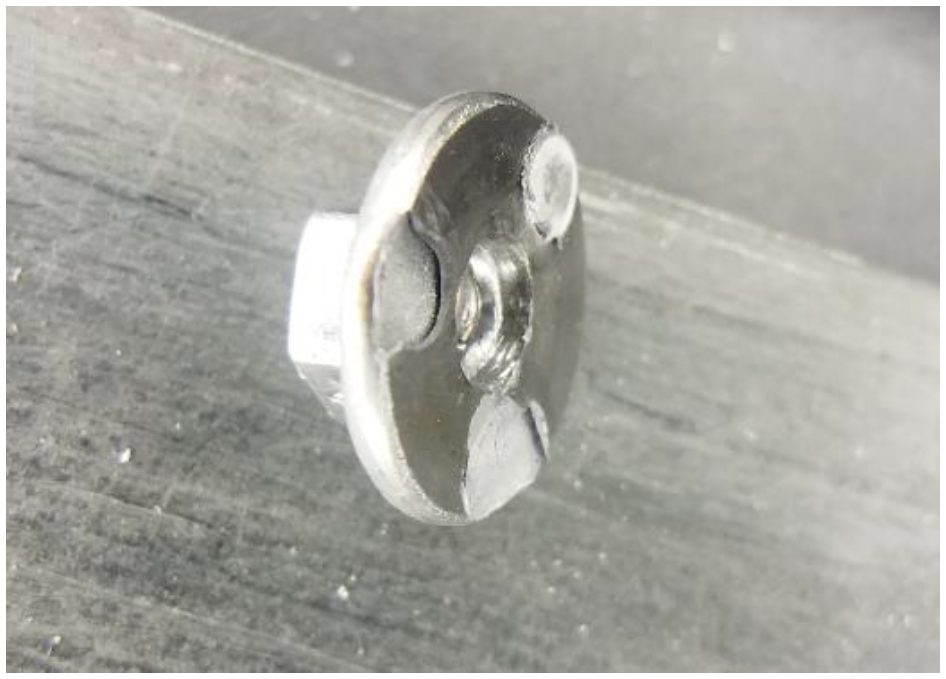
Figure 6: Method B (35.0 kA, 14 ms, 2500 lb.) Common Failure Mode.C-11
Figure 7 shows the microhardness traverse results for both Methods A and B. Method A yields a much larger HAZ. Method B shows a much smaller HAZ, less impact on the base material, and appears to be a solid state bond.
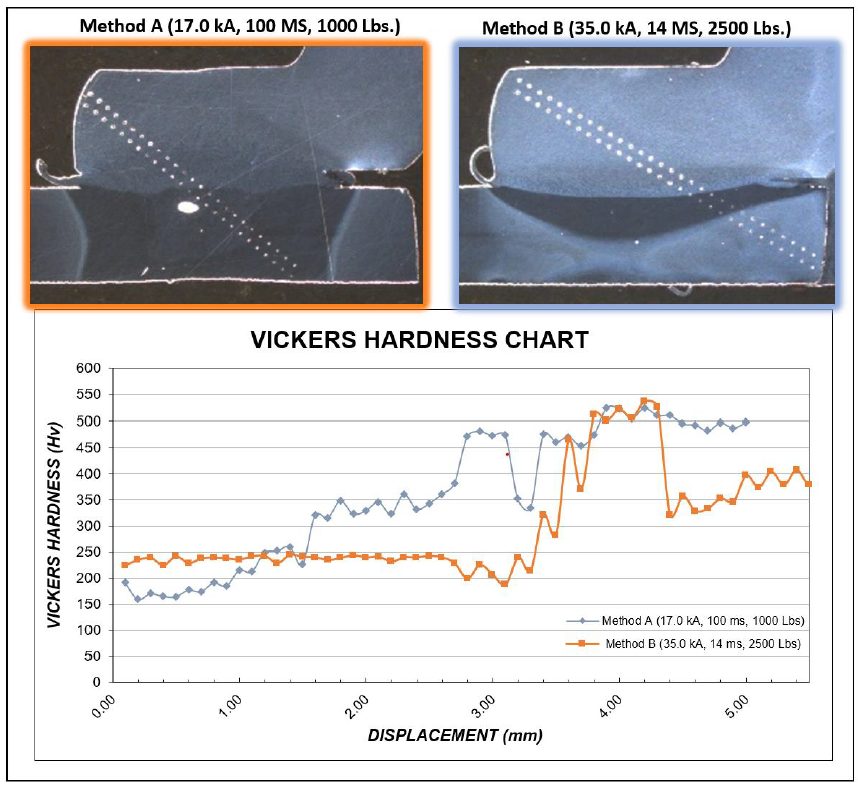
Figure 7: Microhardness Traverse.C-11
Projection Welding
This article summarizes a paper entitled, “Weld Quality Study of Projection Nut Welding with Modular Weld Head”, by F. Jiao, et al.F-7
The study investigates the weld quality of projection nut welding with a patent pending “quick release” modular weld head.
The weld nuts used in the study were 3 projection hex-flanged M6 weld nuts. Operating weld currents ranged from 26-32 kA. The welding time was 5 cycles and the applied electrode force was 450 lb. Figure 1 shows the set up for each test. Figure 2 shows the disassembled parts of the quick release modular weld head. Table 1 lists the material properties of the weld faces.
![Figure 1: Projection Nut Welding Set-Up [(a) with regular weld head, (b) with quick-release modular head, and (c) welding parts set up].](https://ahssinsights.org/wp-content/uploads/2020/07/3122_fig1.jpg)
Figure 1: Projection Nut Welding Set-Up [(a) with regular weld head, (b) with quick-release modular head, and (c) welding parts set up].F-7
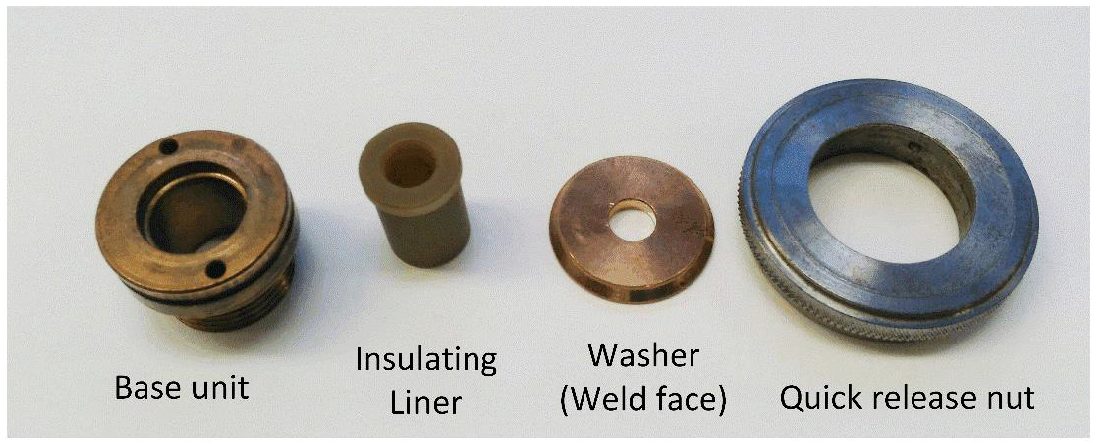
Figure 2: Disassembled Parts of Patent-Pending Quick-Release Modular Weld Head.F-7

Table 1: Material Properties of Weld Faces.F-7
Figure 3 shows the cross section images of the projection welds. Both welds made a weld with 100-micron penetration. The weld made with the regular head had a slightly deeper HAZ as indicated by the dashed line. Figure 4 shows the microhardness profile of both projection welds. Generally, the hardness profile of the welds is quite similar.
![Figure 3: Optical Microscope Images of the Projection Welds After Etching [(a) with regular head (b) with modular head].](https://ahssinsights.org/wp-content/uploads/2020/07/3122_fig3.jpg)
Figure 3: Optical Microscope Images of the Projection Welds After Etching [(a) with regular head (b) with modular head].F-7
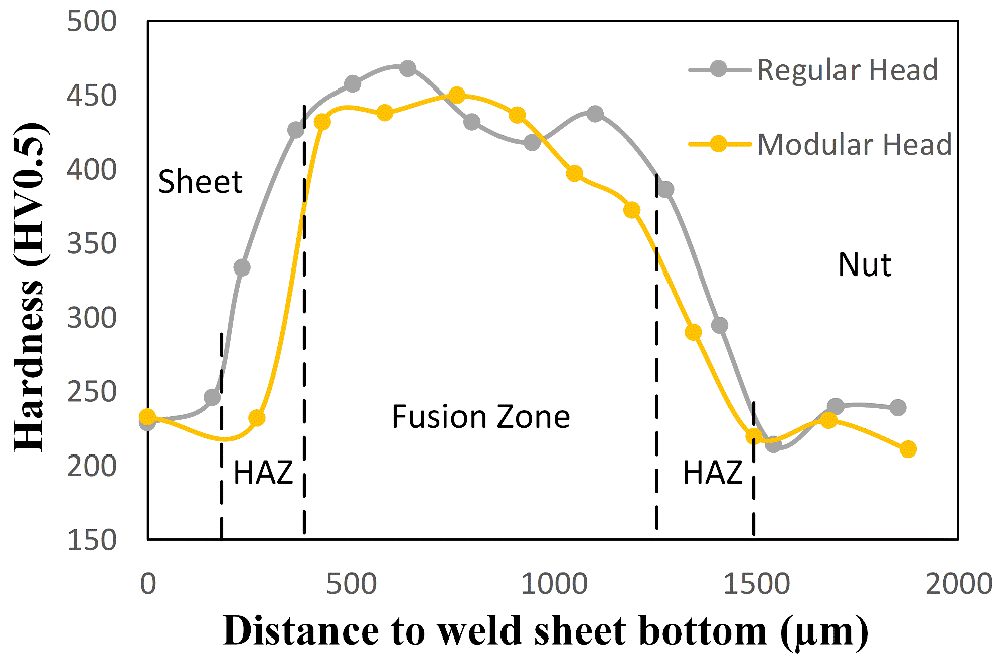
Figure 4: Microhardness of the Projection-Welded Sample with Different Weld Heads.F-7
Figure 5 shows the results of tensile testing on both projection welds. The fracture load with the modular head is higher than that with the regular head at 26 kA. At other currents, the fracture load is very similar.
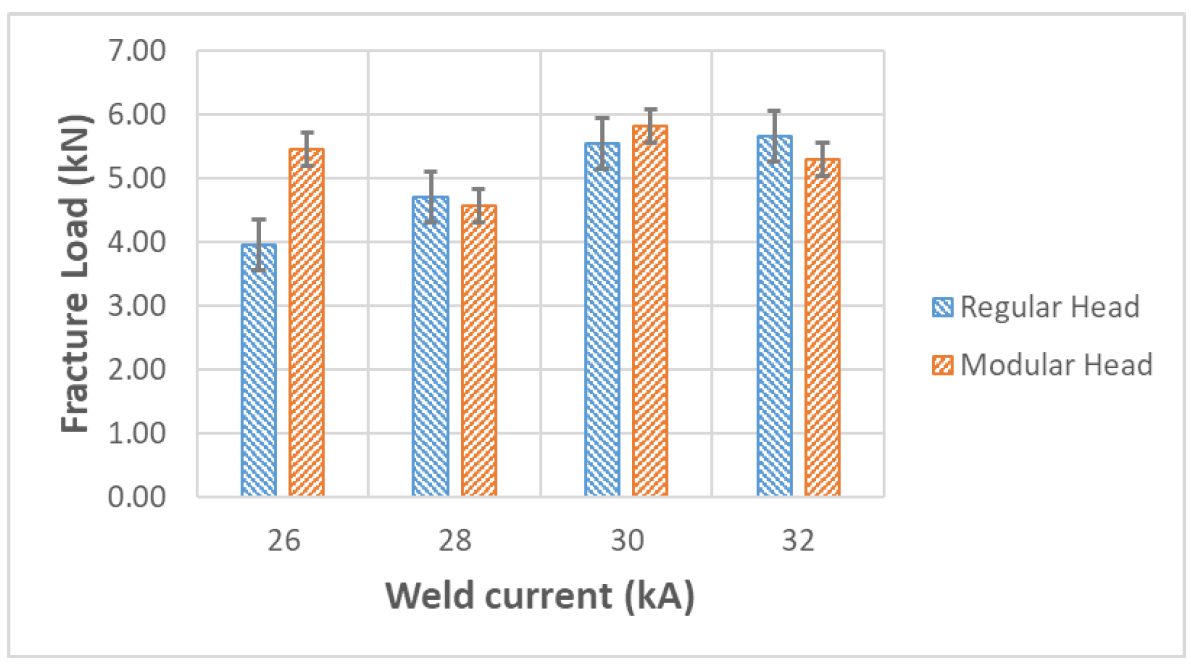
Figure 5: Variation of Fracture Load with Weld Current for Different Weld Head.F-7
Figure 6 shows the weld thickness difference between the modular head and the regular head. The results indicate the weld performance of both heads is very similar up to 1000 welds.
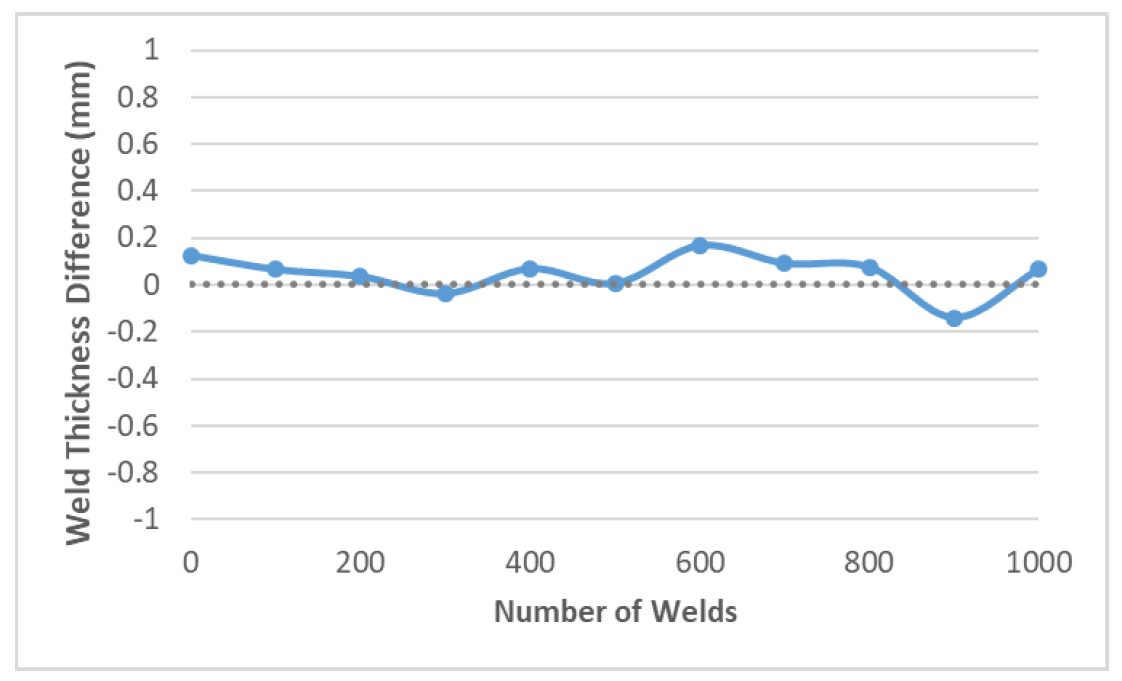
Figure 6: Variation of Weld Thickness Difference Between Regular Head and Modular Head.F-7