Joining
The unique physical characteristics of Advanced High Strength Steels (AHSS) present some challenges to welding and bonding processes. AHSS differ from mild steels by chemical composition and microstructure, and it’s important to note that their microstructures will change from welding operations. For example, intensive localized heat associated with some welding processes causes a significant change in the local microstructure, and hence affect properties. Due to fast Cooling Rates (CR) typical in welding, it is normal to see martensite and/or bainite microstructures in the weld metal and in the Heat-Affected Zone (HAZ).
When joining AHSS, production process control is important for successful assembly. Manufacturers with highly developed joining control methodology will experience no major change in their operations. Others may require additional checks and maintenance. In certain instances, modifications to equipment or processing methodologies may be required for successful joining of AHSS.
The coating methods for AHSS are similar to those for mild steels. Welding of either AHSS or mild steels with coatings will generate fumes. The amount and nature of fumes will depend on the coating thickness, coating composition, joining method, and fillers used to join these materials. The fumes may contain some pollutants. The chemical composition of fumes and the relevant exhaust equipment must meet appropriate regulatory standards. Thicker coatings and higher heat inputs cause more fumes. Additional exhaust systems should be installed. While welding AHSS (with or without metallic or organic coatings and oiled or not oiled) gases and weld fumes are created similar to mild steels. The allowed fumes or gases must comply with respective national rules and regulations.
Joining Processes
Considering recent developments of hybrid approaches to welding, there are now over 100 types of welding processes available for the manufacturer or fabricator to choose from. The reason that there are so many processes is that each process has its list of advantages and disadvantages that make it more or less appropriate for a given application. Arc welding processes offer advantages such as portability and low cost but are relatively slow and use a considerable amount of heating to produce the weld. High energy density processes such as laser welding generally produce low heat inputs and fast welding speeds, but the equipment is very expensive and joint fit-up needs to be ideal. Solid-state welding processes avoid many of the weld discontinuities produced by those requiring melting (fusion), but they may be expensive and often are restricted to limited joint designs. Resistance welding processes are typically very fast and require no additional filler materials but are often limited to thin sheet applications or very high-production applications such as in the automotive industry.
Most welding processes produce a weld (metallic bond) using some combination of heat, time, and/or pressure. Those that rely on extreme heat at the source such as arc and high energy density processes generally need no pressure and relatively small-to-medium amounts of time. This section introduces joining processes that are applicable to HSS automotive applications, while describing unique process attributes.
Solid State Welding Steel to Aluminium
Introduction
Multi material design approach is the most common trend being followed by various industries including the automotive and aerospace industry. The main motive behind the concept is to attain weight reduction and increase the overall efficiency of the system.G-13 Once such desirable combination in the automotive industry is aluminum and steel. Resistance spot welding is one of the most common method used for the joining of aluminum and steel, however, is associated with challenges due to differences in physical properties, mechanical properties, and metallurgical incompatibilities. The inherent aluminum oxide layer creates uncertainties during the process and reduce the electrode life.P-10 Recent trends have shown an increase in the usage of the solid-state processes to attain better weldability between aluminum and steel. Solid state joining processes can be classified into two main categories i.e. mechanical joining processes and solid-state welding.
Mechanical joining involves clinching, clinch riveting, hemming and seaming, blind riveting, self-pierce riveting (SPR), solid pouch riveting, high speed tacking and flow drill screws. Joining is attained via plastic deformation at room temperature. The main advantages of mechanical joining processes involve high tool life, simple equipment, and relative shorter process time. Drawbacks include access required from both sides, bulge and indents, and relatively high amounts of force required during the process.
Solid state welding (SSW) consists of joining processes in which coalescence is attained via pressure or combination of pressure and heat. The peak temperature during the solid-state welding process remains below the melting point and mostly no filler material is required. SSW includes ultrasonic welding, friction welding, and diffusion bonding.
Mechanical Joining
Clinching
Clinching is a local hemming process that includes offsetting, upsetting and flow pressing. In a work carried out by Abe and coworkers, machinal clinching process is investigated for the joining between aluminum and sheets.A-34 Punch and dies with various depths were used to control the material flow and interlocking distance. The main objective was to attain mechanical interlocking without rupture in the sheets. Figure 1a shows the clinching process steps and Figure 1b shows the stress distribution of the formed joint after the clinching process. Figure 1c shows the relationship between interlocking distance and tensile strength of the sheets. Higher strength steel sheets have shown less interlocking due to more resistance to deformation. At higher tool depth the cracks can be seen in the bottom sheets due to excessive plastic deformation (Figure 1d). For all the tests between aluminum and steels, joint was fractured on the aluminum side. Higher strength steels sheets have shown lower cross tension strength due to reduction in the interlocking. Cracks formed during the process can be minimized by eliminating the groove of the die.
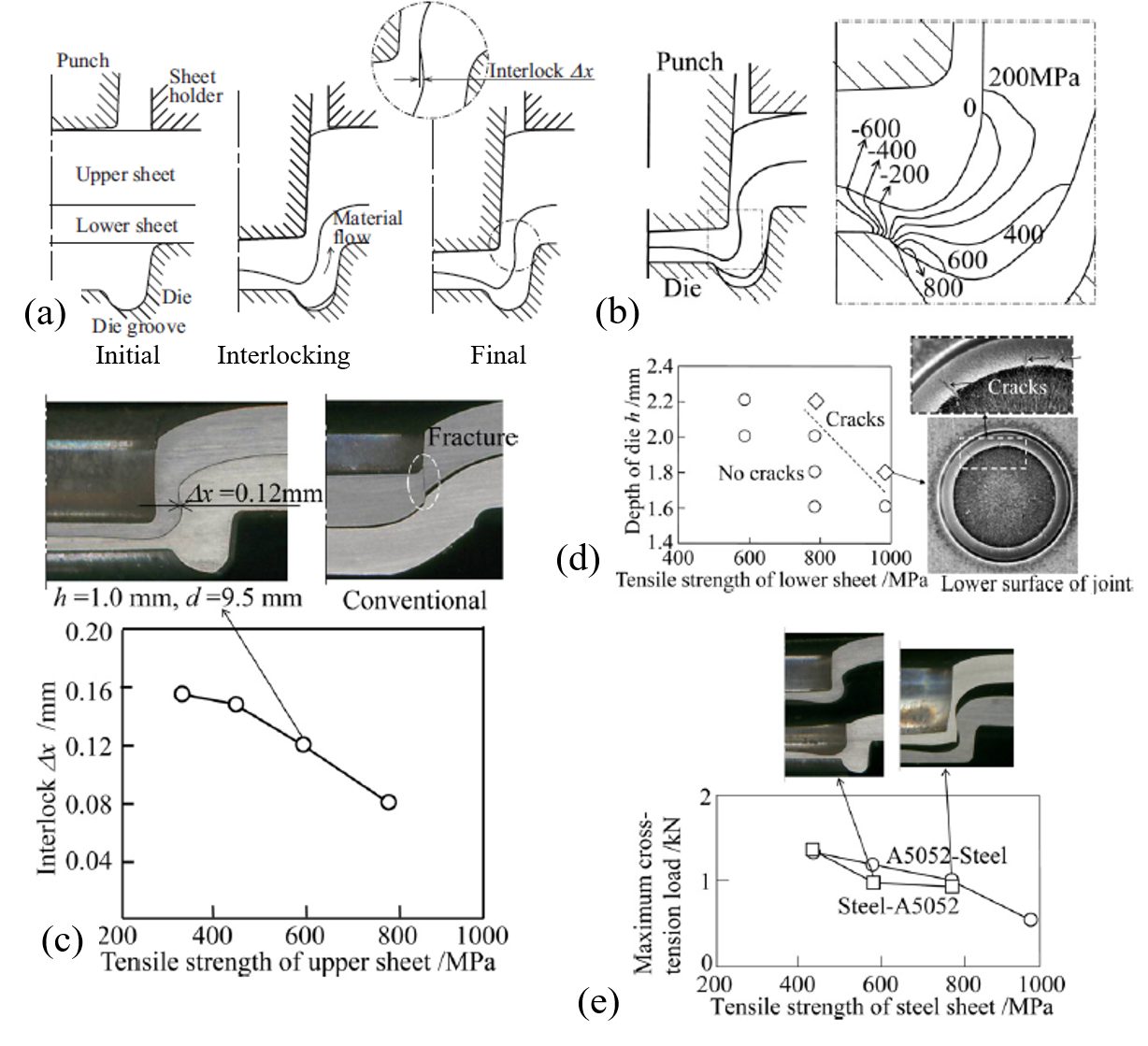
Figure 1: (a) Joining of sheets by mechanical clinching. (b) calculated hydrostatic stress. (c) Relationship between interlock and tensile strength of upper high strength steel obtained from experiment. (d) Occurrence of cracks for lower high strength steel sheets obtained from experiment for d = 8 mm. (e) Maximum load measured from cross-tension test of joined high strength steel and aluminum alloy sheets.A-34
Self-Pierce Riveting
Self-pierce riveting (SPR) is a cold joining process in which two or more sheets are joined via inserting rivet from the top sheets towards bottom sheets and is locked by a die shape. It is one of the most widely used mechanical joining process for the dissimilar materials combination i.e. steel and aluminum. Main advantages include no predrilled holes, no spark, no fumes, and no surface treatment. It comprises of four stages clamping, piercing, flaring, and releasing as shown in Figure 2 (a & b). Figure 2c shows the semi tubular and full tubular rivet through the multi materials stacks. The use of tubular or semi tubular rivet depends upon the material stacks combination. Steel rivets have shown higher mechanical strength compared to aluminum rivets (Figure 2d). The right half of the Figure 2e shows possible defects occur during riveting process.
![Figure 2: (a) A schematic diagram of the four stages of a SPR process, L-16 (b) A typical four-stage force–displacement curve of an SPR process, L-16 (c) Thick stacks with a semitubular SPR rivet and a fully tubular SPR rivet, L-16 (d) Comparison of the single-joint strength between aluminum rivets and a steel rivet with combined shearing and pull-out loading with loading angle α = 45°, H-11 (e) SPR joint quality and some faults [4]& (f) The displacement-load curves of the joints with different stack orientations. S-31](https://ahssinsights.org/wp-content/uploads/2020/09/image003.jpg)
Figure 2: (a) A schematic diagram of the four stages of a SPR process,L-16 (b) A typical four-stage force–displacement curve of an SPR process,L-16 (c) Thick stacks with a semitubular SPR rivet and a fully tubular SPR rivet,L-16 (d) Comparison of the single-joint strength between aluminum rivets and a steel rivet with combined shearing and pull-out loading with loading angle α = 45°,H-11 (e) SPR joint quality and some faults,L-16 and (f) The displacement-load curves of the joints with different stack orientations.S-31
Three main aspects are considered to determine the joint quality i.e. rivet head height, interlock distance and minimum remaining bottom material. Han et al. H-12 concluded that lower head height could result in higher interlocking distance for joining aluminum and steel alloys. Sun et al. S-31 has evaluated different stack configuration for the 5128O aluminum alloy with the HSLA 1350 alloys as shown in Figure 2f. It was concluded in the study that high strength materials such as HSLA 1350 should be on the tail side as it promotes sufficient interlocking especially when it comes to join thicker sheets (>3 mm).
Flow Drill Screw Process
Flow drill screw process is a thermomechanical joining process, combining friction drilling and thread forming process. During the process, screws act as fasteners, drilling and tapping tools. A main advantage is that only single sided access is required for flow drill screw (FDS) process. FDS can be used with or without drilling the hole depending upon the screw material and stack materials.
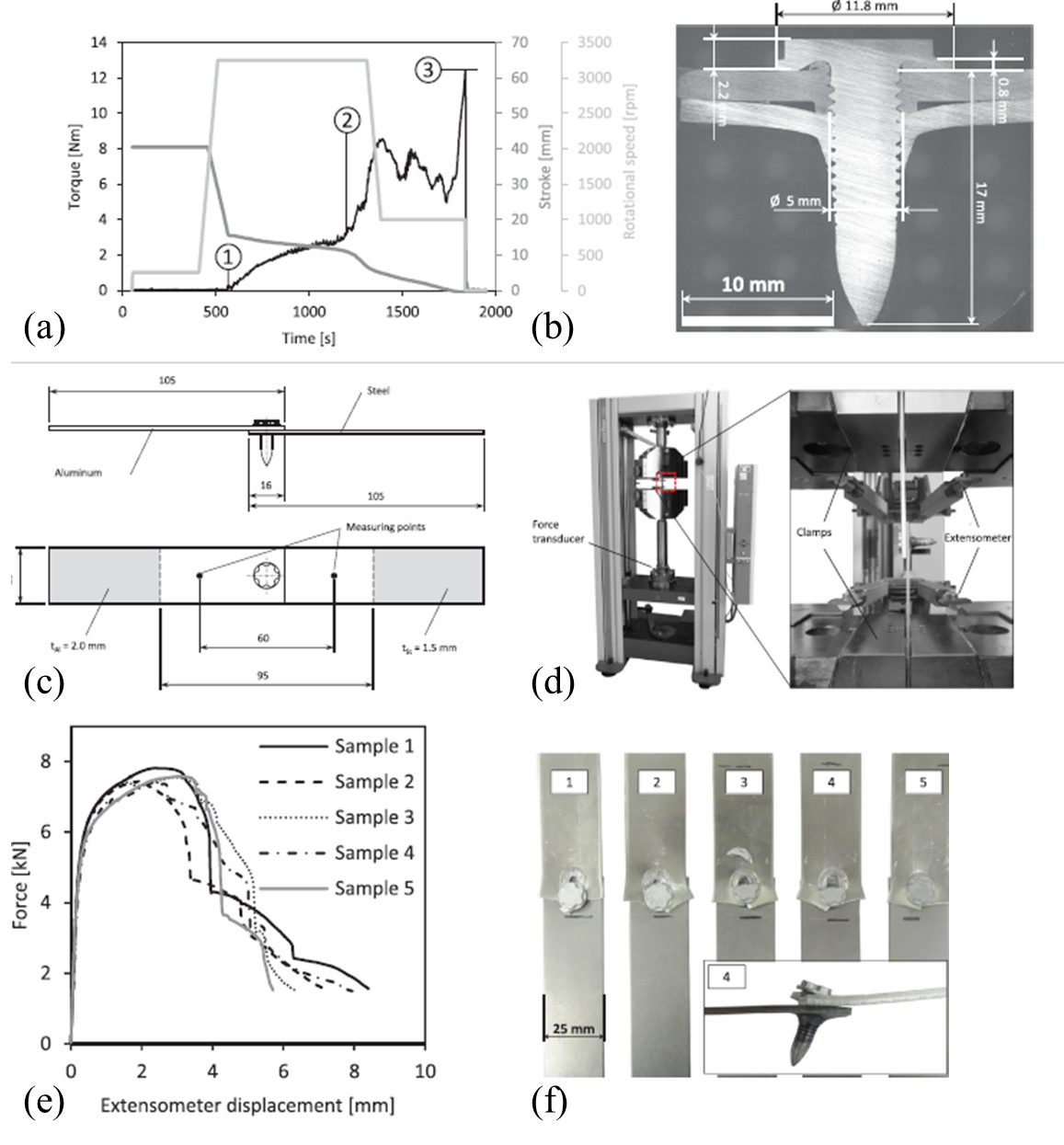
Figure 3: Joining parameters and cross section of single-lap shear specimen. (a) Relevant screwing parameters over time (representative example): start of warming up and penetration of the substrate material, beginning of thread forming, tightening torque. (b) Cross-section of connection with nominal dimensions. Static single-lap shear test of an aluminum-steel flow drill connection. (c) Geometry of specimen. (d) Experimental setup. (e) Force-displacement curves. (f) Fracture appearance.G-15
Graff and coworkers have evaluated the flow stress during FDS process between aluminum and steel.G-15 A 5083H111 aluminum alloy and HC340LA micro alloyed steel were used in this study. Figure 3a shows the experimental parameters and Figure 3b shows the cross section of the formed joint. Samples were fractured in the aluminum side each time with the peak failure load of 7 kN.
Solid State Welding, Steel to Aluminium
Ultrasonic Welding
In ultrasonic metal welding process, joining is achieved via shear motion at the faying surfaces of the materials to be joined. It is combination of friction and cold pressure welding. This process is more ideal in joining thinner sheets of aluminum and steel. In a technical report published by Shakil and coworkers, ultrasonic metal welding between 3003 Al alloy (thickness 50 µm) and 304 stainless steel (thickness 300 µm) was evaluated.S-32 Figure 4 shows the schematics of their ultrasonic metal welding process. In this work, a 2.4 kW lateral drive ultrasonic welder with a welding tip of 8 x 6 mm and 25 mm overlapping between the sheets without any surface preparation was utilized. Welding energy was varied between 75 J and 200 J and clamping force of 30, 40, 50, and 60 psi was utilized. It is shown in the studies that increase in the clamping force decreases the weld time. It is attributed to more heat generation due to increased sliding resistance.

Figure 4: (a) Schematic diagram of lateral derive system. (b) Weld sample geometry.S-32
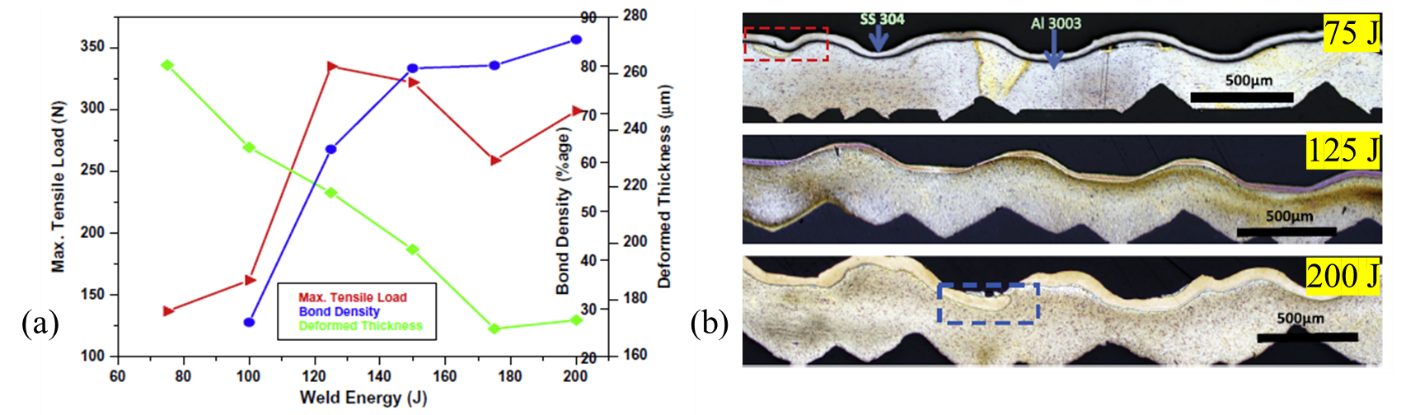
Figure 5: (a) Correlation of weld performance with bond density and deformed thickness. (b) Optical micrographs of 3003 Al and 304 SS weld cross-section produced with a pressure of 40 psi with increasing weld energies.S-32
A 40 psi maximum peak load to failure is observed at an energy input of 125 J as shown in the Figure 5. Optical micrographs revealed the wave like bonding line at the interface due to continuous shear oscillations. The joining process has been summarized into three steps i.e. formation of the micro bonds at the interface due to expansion followed by continuous deformation due to normal and shear oscillations at the faying surfaces. Lastly, these micro bonds grow and join to form a fully bonded interface. Moreover, it is specified that for a good quality weld, good combination of the bond density and material deformation is required.
In another work carried out by Jiromaru and coworkers, butt welding of thicker specimens (6 mm thick 5052 Al alloy and 304 stainless steel) via ultrasonic welding system (50 kW) of 15 kHz is evaluated.T-16 Figure 6 shows the schematics of the process. Welds were obtained at various amplitudes i.e. the peak to zero vibration is varied between 17 and 27 µm, static pressure of 20 Mpa and for a duration of 2 seconds. Figure 6 shows the schematics of the ultrasonic butt-welding system. Static and hydraulic pressure were utilized to clamp the welding specimens. Burrs were observed on the aluminum side and is attributed to the lower hardness of the aluminum compared to steel. Maximum strength attained was around 100 MPa at various amplitudes as shown in Figure 7. Significant decrease in the hardness is reported near the aluminum side and increase in the hardness is observed 2 mm away from the weld line on the stainless-steel side. The hardness value is lower in the upper and lower region compared to center region due to conduction. 20 % variation was observed in the hardness profile through the cross section of the welded specimens from top to bottom.
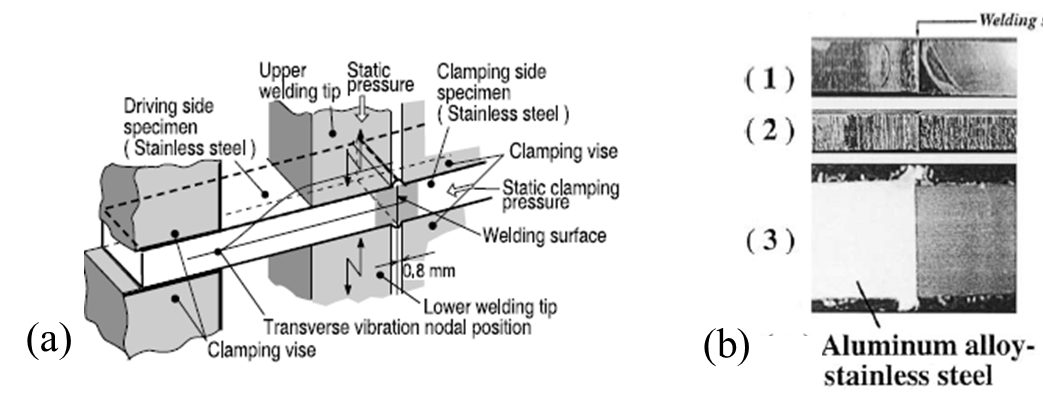
Figure 6: (a)) 15 kHz ultrasonic butt welding equipment using an upper and a lower welding tips, and hydraulic vices for clamping welding specimens and inducing static pressure to welding surface. (b); Welded conditions of 6-mm-thick, 10-mm-wide stainless steel plate specimens. (1) Upper view, (2) side view and (3) cross-section of welded 6-mm-thick, 10-mm.T-16
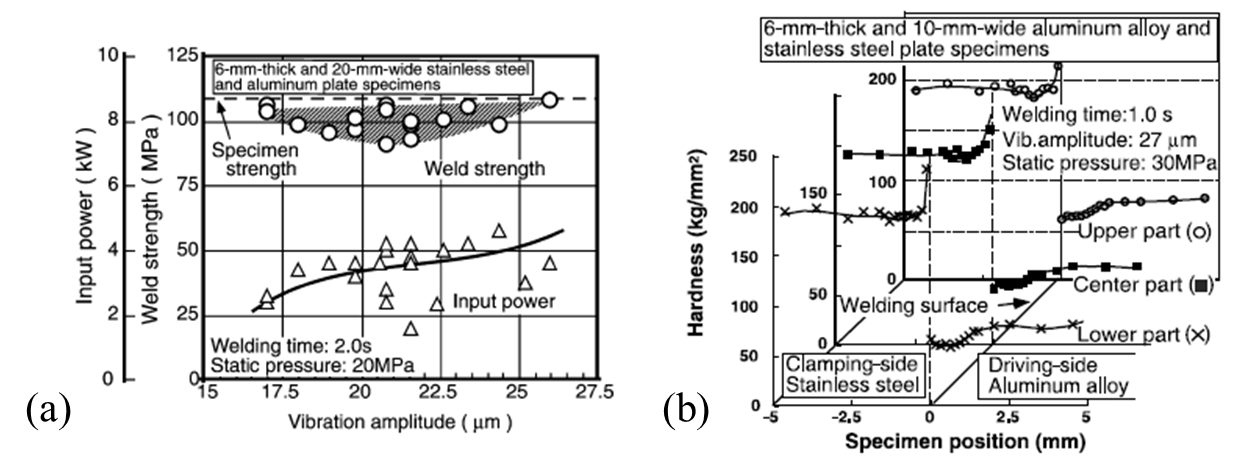
Figure 7: (a) Relationship between vibration amplitude, input power and weld strength of 6.0-mm-thick and 20-mm-wide pure aluminum and electrolytically polished stainless steel plate specimens. (b) Hardness tributions along a cross-section of a 6.0-mm-thick and 10-mm-wide welded aluminum alloy–stainless steel specimen at upper, center, lower parts. Welding time: 1.0 s.T-16
Friction Welding
In friction welding, joining is attained under the application of the pressure and rotation i.e. torque is applied and the materials to be joined are pushed against each other. Main advantages include reproducibility, shorter time, no filler material, and high-quality welds. However high capital cost is a limitation of this process. Friction welding processes involve several methods, such as linear friction welding, rotary friction welding and friction stir welding. Fukumoto and coworkers have evaluated the friction welding of 1050 aluminum alloy to austenitic stainless steel.F-8 Figure 8 shows the schematics of the materials to be joined. This study was carried out at constant parameters of 2400 rev/min, frictional pressure of 50 MPa, forging pressure of 150 MPa, and forging time of 6 seconds. However, frictional time was varied between 0.1 to 2 seconds. Flash was found to be symmetrical around the weld. Smaller heat affected zone was observed on the steel side. Intermetallic compounds Fe2Al5, Fe3Al and FeAl were detected in the reaction layer. Reaction layer thickness was found to be around 500 nm as shown in the Figure 9.
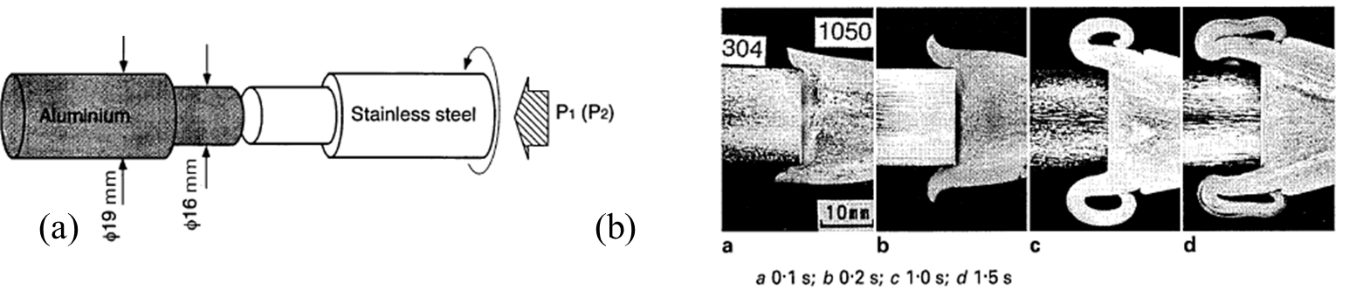
Figure 8: (a) Schematic illustration of friction welding procedure. (b) Cross-sectional macrostructures of friction welded joints for given frictional time t.F-8
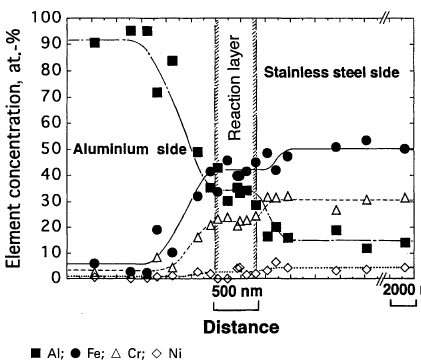
Figure 9: EDS analysis along line along the interface between aluminum and steel at 1.5 seconds.F-8
In another work carried out by Matsuda and coworkers, high frequency linear friction welding between aluminum alloys (5083 & 6063) and 304 stainless steel alloys was evaluated.M-14 The friction frequency was 245 Hz with an amplitude of 1.8 mm. It can be seen in the Figure 10 that loading ratio and friction time influences the tensile strength of the joints. Loading ratio was calculated as a function of proof stress with respect to aluminum alloys and was found to be 145 MPa. Maximum strength was reported at 60 % loading ratio at a friction time of 1 second i.e. 300 MPa.
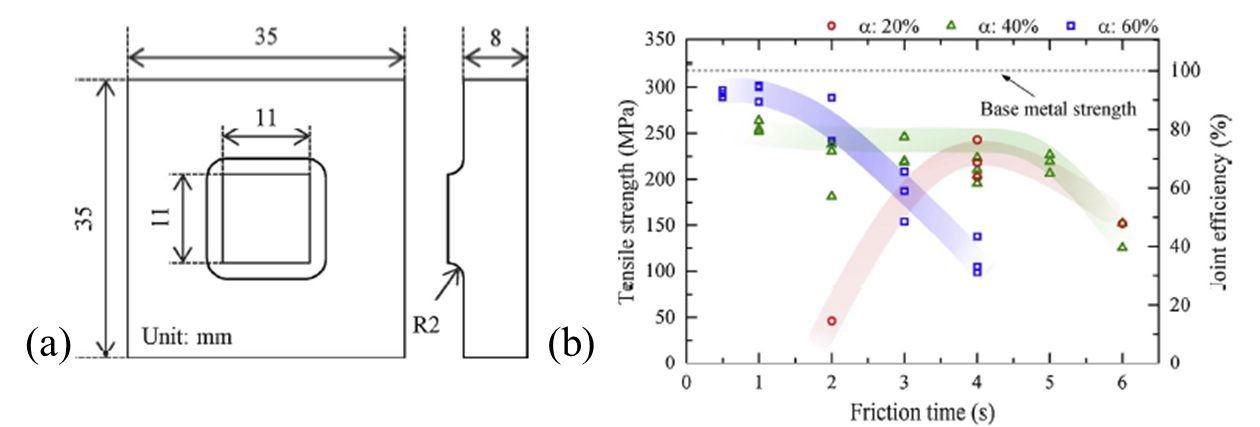
Figure 10: (a) Schematic illustration of the specimen. (b) Influence of loading ratio α and friction time on the tensile strength of Al-5083/304 SS.M-14
Tensile strength of these joints decreases with the increase in the friction time. Longer time promotes to wider reaction layer that is detrimental for the weld quality. Figure 11 shows the cross-sectional SEM images between Al5083/SS304 at 40 % and 60 % loading ratio. At longer friction times, cracks and fractures were observed at the interface. No reaction layer was seen at 20 % loading ratio. Reaction layer between Al5083/SS304 decreases below 500 nm at higher pressure and at longer time cracks were observed due to fracture of the intermetallic compounds (Figure 11).
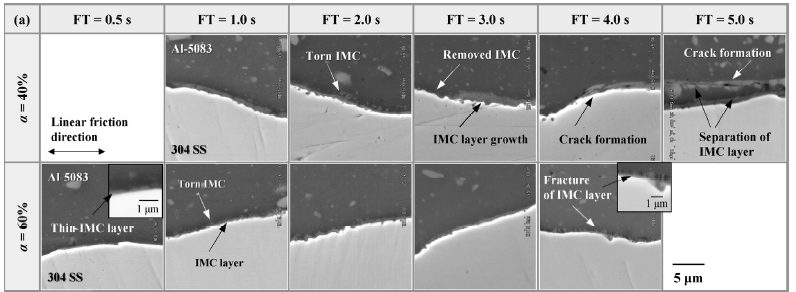
Figure 11: Cross-sectional SEM images of (a) Al-5083/304 SS joints showing the temporal evolution of their interfacial structures (FT=friction time)M-14
Kimapong and T. Watanabe reported butt joint of an aluminum alloy and mild steel via friction stir welding process.W-17 Up to 86 % of the joint strength is attained compared to aluminum alloy. SS400 mild steel plates A5083 plates having a thickness of 2 mm was used in this study. Figure 12a shows the schematics of the experimental procedure. A high-speed steel tools with 15 mm shoulder and 2 mm threaded pin diameter was used in this study. Rotational speed was varied from 100 to 1250 rpm. The pin traverse speed was 25 mm/min. Figure 12 (c&d) shows the effect of the rotating speed on the weldability of the friction stir welding process. Figure 12e shows the tensile strength of 240 MPa at a rotation speed of 250 rpm. At lower rotational speeds, the pin wore out due to inadequate heat generation and at higher rotational speed oxidation occurred due to the presence of aluminum and magnesium. Lower rotation speeds do not generate enough heat required for plasticization whereas higher rotational speeds lead to higher temperature that promotes the oxidation of the aluminum and magnesium, hence decrease the quality of the weld. It is therefore important to select right rotational speed for the sound weld.
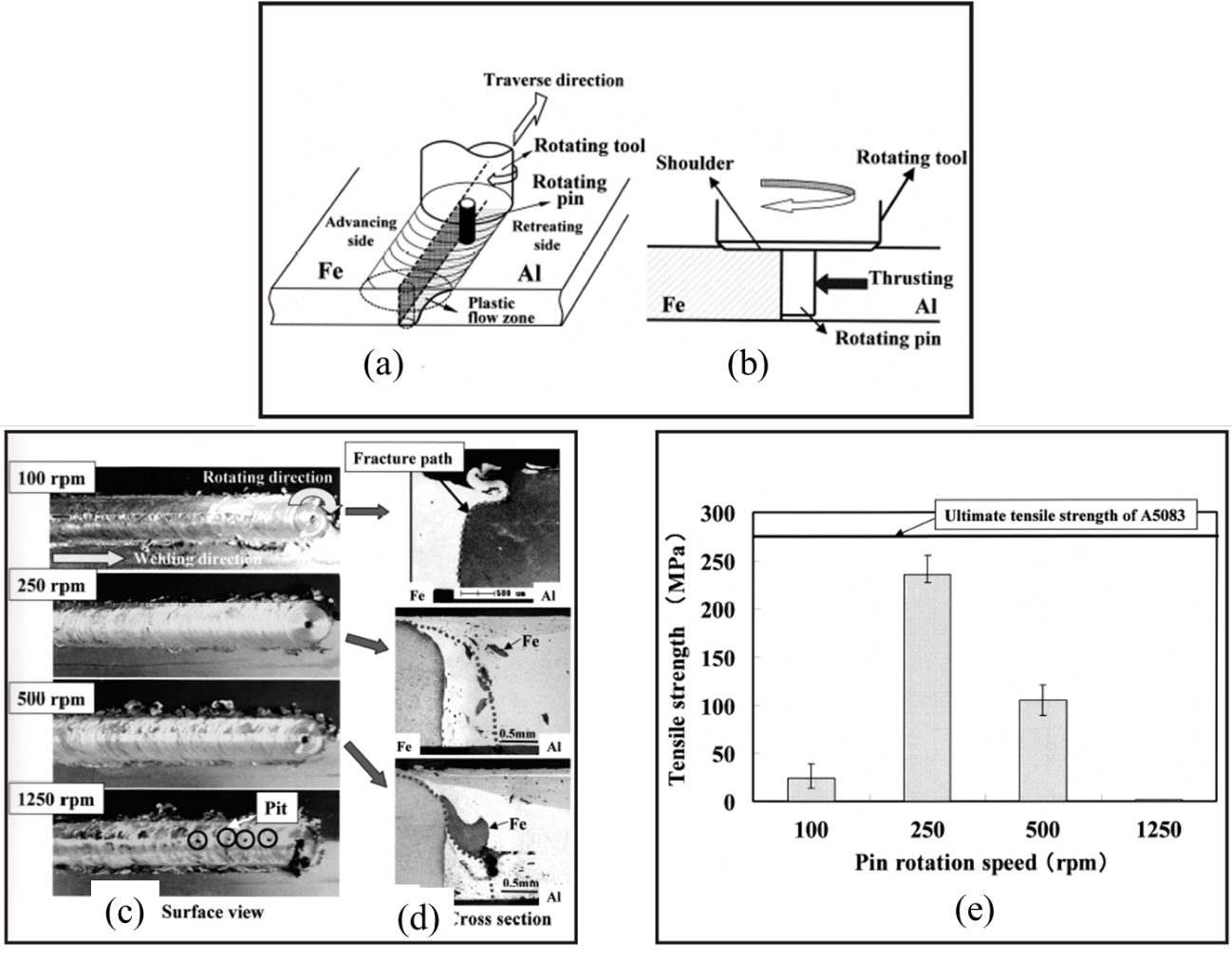
Figure 12: Schematic of the rotating pin position in this study: (a) Bird’s eye view of the method; (b) view of the cross section perpendicular to the weld interface. Effects of pin rotation speed; (c) surface view; (d) cross-sectional structure with the fracture path indicated by a dashed line in the welds. (e) Relation between pin rotation speed and joint tensile strength.W-17
In an investigation carried out by K. Chen, the relationship between axial plunge force and process temperature during friction stir spot welding of the Al6061 with TRIP 780/800 steel is evaluated.C-12 It was reported that increase in the plunge speed leads to larger reaction forces and lower peak temperature as shown in Figure 13. The bonding mechanisms during the process involve, mechanical interlock and chemical bonding. Moreover, it was reported that Zn coating can enhance the joint quality during friction stir spot welding process.
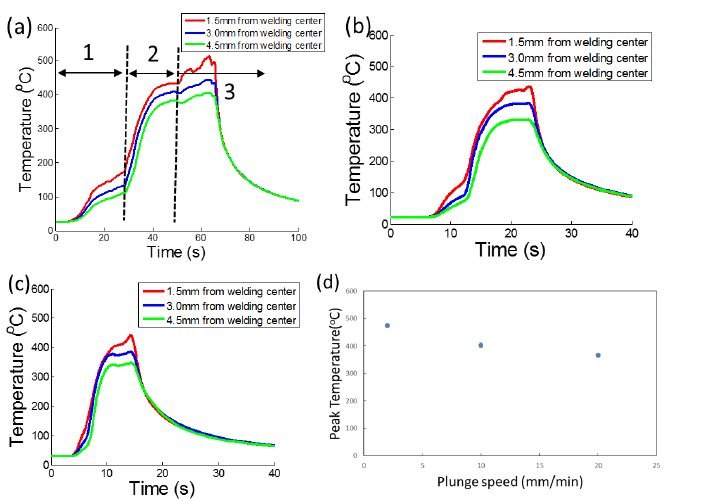
Figure 13: Temperature profile with different plunge speeds: (a) 2 mm/min, (b) 10 mm/min, (c) 20 mm/min, and (d) peak temperature with different plunge speeds).C-12
Diffusion Bonding
In diffusion bonding, sheets to be joined are placed against each other under a vacuum or an inert gas. At the faying surfaces, bonding is attained via diffusion due to difference in the chemical concentration. Diffusion bonding process can be accelerated by operating the process at higher temperatures. This process is not suitable for the direct joining of the aluminum and steel, however with assistance of interlayer a sound weld can be obtained via this process. Bhanumurthy has used interlayer in the Al/Zn/Cu/Ag–Ag/Cu/Ni/SS interlayer pattern to minimize metallurgical incompatibilities for the joining of the aluminum alloys with the stainless steels.B-7 All the layers were deposited electrochemically. 304 stainless steel and pure aluminum (99.8 & pure) were used in this study (10 x 5 x 4 mm). The whole assembly was placed in vacuum (10‑3 Pa) at a heating rate of 300 k h-1. Temperature range of 250 oC to 550 oC and time duration of 1h to 4h under a pressure of 50 MPa was evaluated in this study.
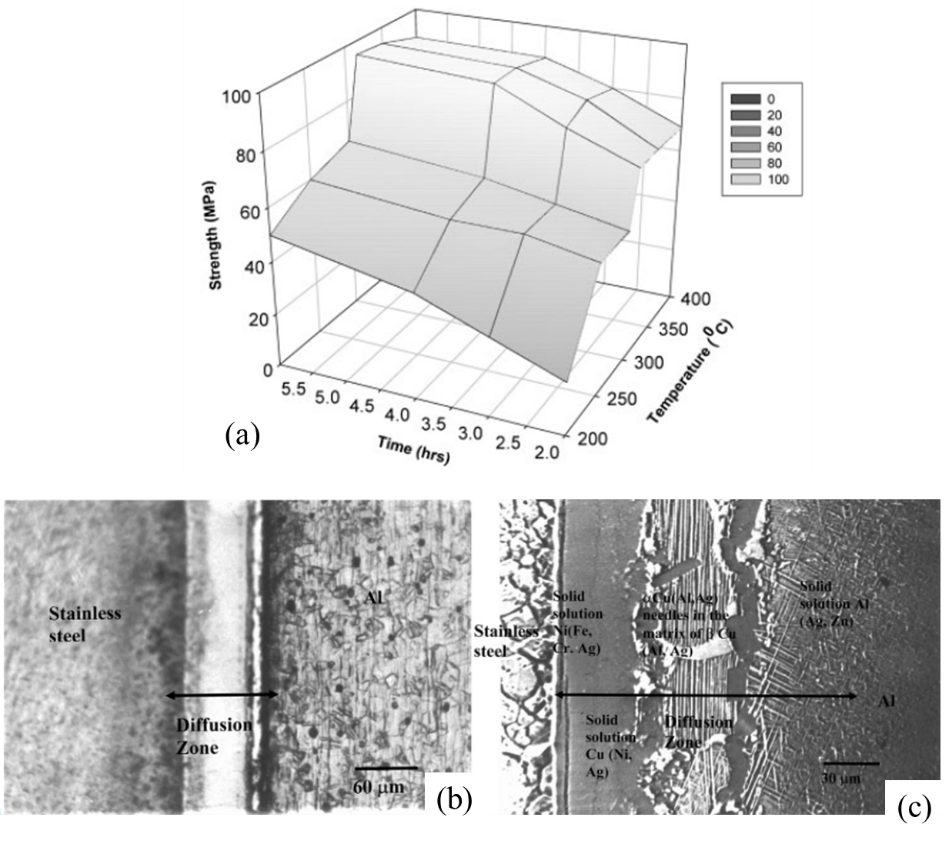
Figure 14: (a) Bond strength data for cylindrical specimens with variation in bonding time and temperature. Optical micrograph for specimen bonded at (b) 450 oC and (c) 550 oC for 4 h. B-7
Diffusion bonding done at 315 oC for 4 h have shown maximum strength of ~90 MPa. Specimens bonded at 300 oC failed at the Ag/Ag interface. Maximum diffusion width was 19 µm at 350 oC for 4 hours. Overall, the diffusion bonding process was found to be a strong function of the surface preparation and diffusivities of the bordering species.
Resistance Spot Welding
In a comprehensive analysis carried out by Sigler and Carlson, hindrances occurred between the joining of aluminum and steel alloys via resistance spot welding process and approaches were established to overcome those challenges.S-33 Main challenges include, the difference in the melting temperature, formation of intermetallic compounds and natural oxide layers. 1.2-mm 6022T4 aluminum alloy, 1.0, 1.5- & 2.0-mm low carbon steel sheets were evaluated in this study. Configuration of lap shear test and couch peel test is shown in Figure 15A. Multiring doom electrode patented by General Motors were used in this study. Figure 15B shows the joint formed between 1.2-mm Al6062 aluminum alloy and 1.5 mm steel sheet at 3.6 kN and 14 kA current. Welding times were 200, 400, 600 and 800ms, with 45ms pulse and cool time of 5ms between pulses. Weld performed at 200ms are not shown in this report due to insufficient strength. Both shrinkage and gas porosities were observed at each time condition. At higher time, porosities join together to form bigger porosities and these was no increase in the bond length form 600ms to 800ms.
![Figure 15: (A) Configuration of Lap-Shear and Coach-Peel Mechanical Test Specimens ,(B) Weld Microstructures for a 1.2-mm AA6022-T4 (top sheet) to 1.5-mm HDG LCS (bottom sheet) Stack-Up Welded using MRD Electrodes at 800 lb and 14 kA , (C) Secondary and Back-Scattered Electron Image (BEI) Micrographs [secondary electron images (SEI) left and BEI right] of the Al-Side Weld Fracture Surfaces for 1.2-mm AA6022-T4 to 1.5-mm HDG LCS Stack-Up Welded at 800-lb Clamping Force and 14-kA Welding Current, (D) Coach-Peel and Lap-Shear Mechanical Properties for (a) 1.2-mm AA6022-T4 Welded to 1.0-mm HDG LCS and (b) 1.2-mm AA6022-T4 Welded to 2.0-mm HDG LCS [, & (E) Coach-Peel and Lap-Shear Mechanical Properties for Coupons of Zn-Coated 1.2-mm AA6022-T4 to 1.0-mm HDG LCS Stack-Up Welded at 800 lb, 250 ms, and 16 kA.S-33](https://ahssinsights.org/wp-content/uploads/2020/09/image029.jpg)
Figure 15: (A) Configuration of Lap-Shear and Coach-Peel Mechanical Test Specimens ,(B) Weld Microstructures for a 1.2-mm AA6022-T4 (top sheet) to 1.5-mm HDG LCS (bottom sheet) Stack-Up Welded using MRD Electrodes at 800 lb and 14 kA , (C) Secondary and Back-Scattered Electron Image (BEI) Micrographs [secondary electron images (SEI) left and BEI right] of the Al-Side Weld Fracture Surfaces for 1.2-mm AA6022-T4 to 1.5-mm HDG LCS Stack-Up Welded at 800-lb Clamping Force and 14-kA Welding Current, (D) Coach-Peel and Lap-Shear Mechanical Properties for (a) 1.2-mm AA6022-T4 Welded to 1.0-mm HDG LCS and (b) 1.2-mm AA6022-T4 Welded to 2.0-mm HDG LCS, and (E) Coach-Peel and Lap-Shear Mechanical Properties for Coupons of Zn-Coated 1.2-mm AA6022-T4 to 1.0-mm HDG LCS Stack-Up Welded at 800 lb, 250 ms, and 16 kA.S-33
Figure 15C shows the SEM of the fractured surfaces. At 200ms porosities were star shaped with arms extending towards periphery of the nugget. Increasing time leads to shorter thicker and fewer porosities. It is an indication of the consolidation of porosities towards the center. Here dark grey features are an indication of the aluminum. Figure 15D shows the comparison of lap shear strength at various conditions. Maximum lap shear strength is observed between 1.2-mm aluminum sheet and 2-mm steel sheet i.e. 64% higher than that of aluminum spot welds. Lower mechanical strength is attributed to discontinuities that could be gas and shrinkage porosities. Moreover Figure 15E shows the effect of the Zn coating on the aluminum. Higher peel strength was observed in the presence of the Zinc coatings. It was summarized in this study that the main reason for the poor mechanical properties is shrinkage and gas porosities. Removing aluminum oxide layer and replacing it with zinc layer can help in the elimination of the defects. Moreover, using multiple weld schedules can also improve weld joint quality.
Other investigations carried out at The Ohio State University and Edison Welding Institute, researchers have utilized capacitor discharge power for creating resistance spot welds between aluminum and steel.G-16 The main objective is to minimize the time through which the current is delivered in the sheets to minimize the growth of the intermetallic layer. Figure 16a shows the shape of the current waveform delivered in 15ms. Figure 16b shows the increase in the mechanical strength as a function of increasing current value.
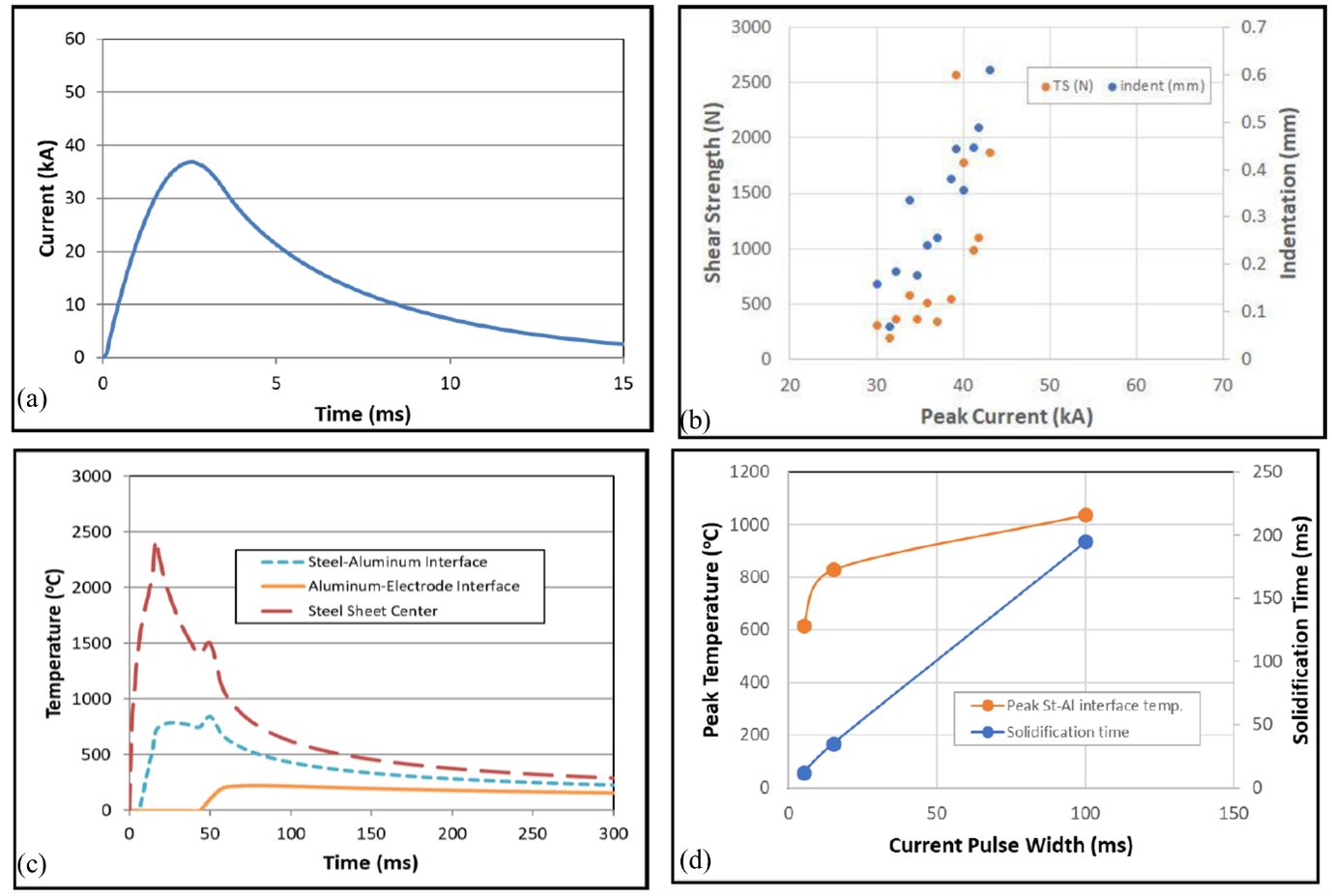
Figure 16: (a) Current waveform for a weld made with the 75:1 turns ratio, (b) Current-range curve for welding with the 75:1 turns ratio ,(c) Estimated thermal profiles for an al-to-steel spot weld made with a 15-ms pulse width & (d) Peak temperature and solidification time for the al weld nuggets as a function of pulse width.G-16
Thermal profiles were measured at multiple interfaces (Figure 16c) and Figure 16d show the variation in the peak temperature as the function of the current pulse width, which increases in direct proportion. Overall, using capacitor discharge power resulted in separate nuggets and narrower pulse widths. At 5ms, 3-5% weld indentation was observed on the aluminum side with no weld discontinuities. Longer weld time takes more solidification time and wider pulse widths resulted in thicker intermetallics layer.
Hybrid Welding Processes
To improve the dissimilar material joining processes, some studies have reported hybrid welding processes. One such work is carried out by J. Gould from Edison Welding Institute, during which resistance welding and linear friction welding process is utilized.G-17 Low force friction welding process is reported and configuration of the setup is based upon the resistance butt welding frame coupled with hydraulic systems to provide linear motion during the process (Figure 17). Figure 17b shows the tensile results carried out at 30 kA and peak strength was observed near 450 MPa with highest number of translations and upset distance (4130-4130 steel sheets). No melting was observed at any of these parameters. For Al6061 aluminum alloy welding parameters were modified as shown in Figure 17c. Linear motion was varied from 2 mm to 4 mm. Peak strength was observed at 4 mm displacement. Strain map was developed for a steel sheet and it shows that to achieve the required amount of strains, significant upset is required during the process. Using this competitive process, less than 1/5 contact pressure without melting is reported compared to conventional process. This hybrid process reduces the frequency and translation required during friction welding process.
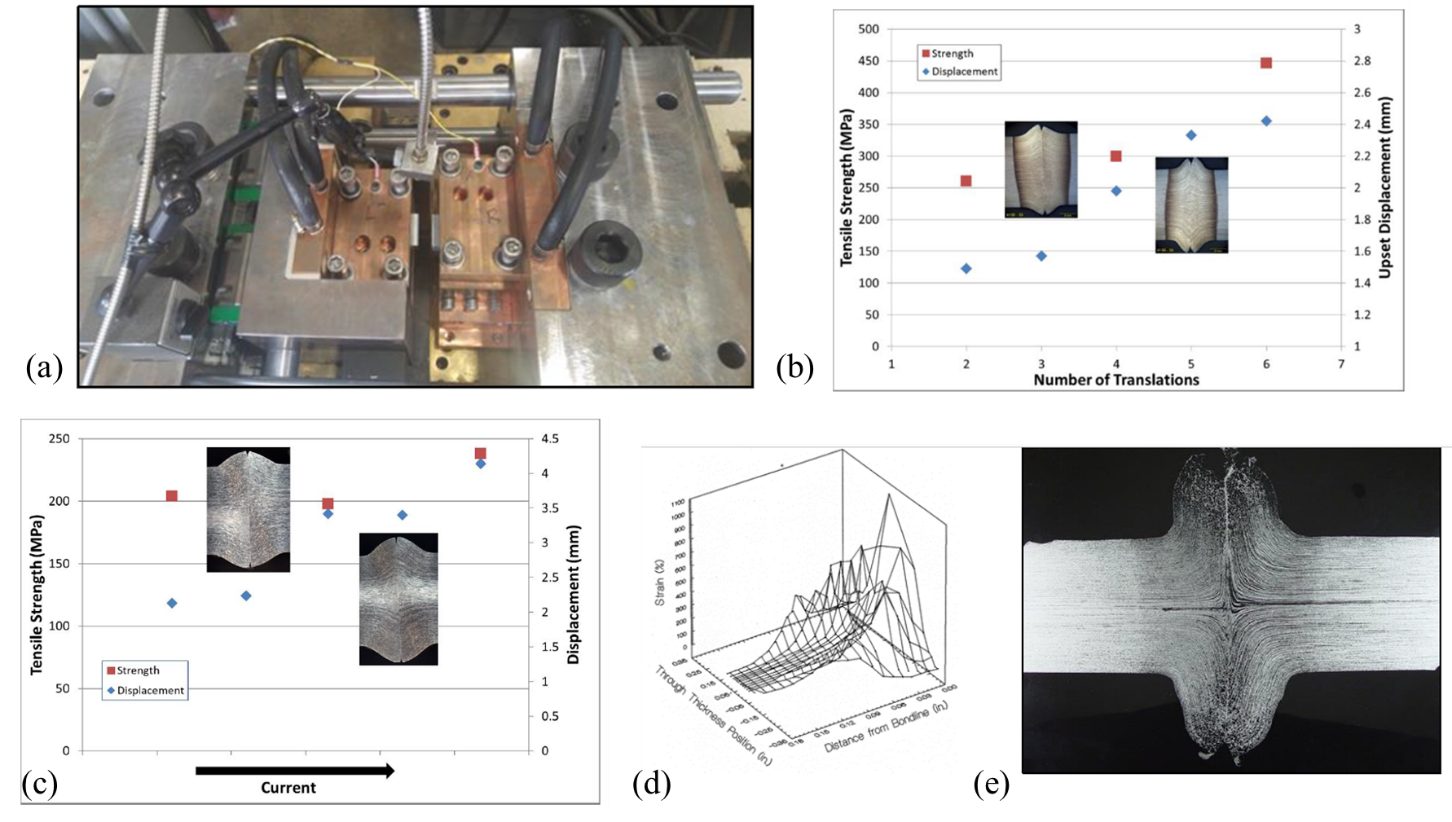
Figure 17: (a) Details of the tooling pack used to accomplish low-force friction welding. (b) Sample process window plot for steel joints made using increasing numbers of translational actions. (c) Process window plot showing variations in aluminum joints made using increasing current. (d) Two-dimensional strain map taken from a flash-butt weld on 6-mm-thick steel. (e) Flash-butt weld cross section corresponding to the strain map.G-17
Y. Lu and co-workers L-17 developed the ultrasonic plus resistance spot welding process (U+RSW). In this process, a thin aluminum sheet was joined with a steel sheet via ultrasonic welding, followed by the RSW between the aluminum side of previously sheet and an additional aluminum sheet as shown in Figure 18. In this study they have used 1-mm-thick AA6061-T6 to 0.9-mm-thick AISI 1008 steel with 0.4-mm-thick AA6061-T6 as the insert.
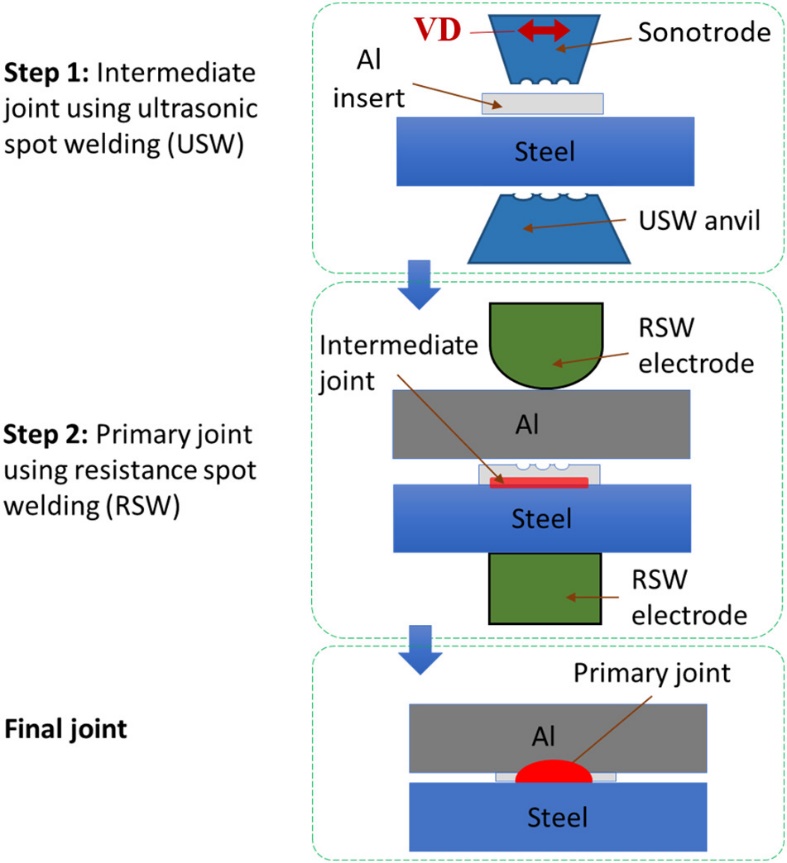
Figure 18: Schematic diagram of U + RSW process. VD is the sonotrode’s vibration direction for USW of intermediate joint.L-17
U+RSW method has shown improved mechanical properties, (Figure 19) tensile shear strength and, energy absorbed prior to failure. It has shown relatively thin intermetallic layer.
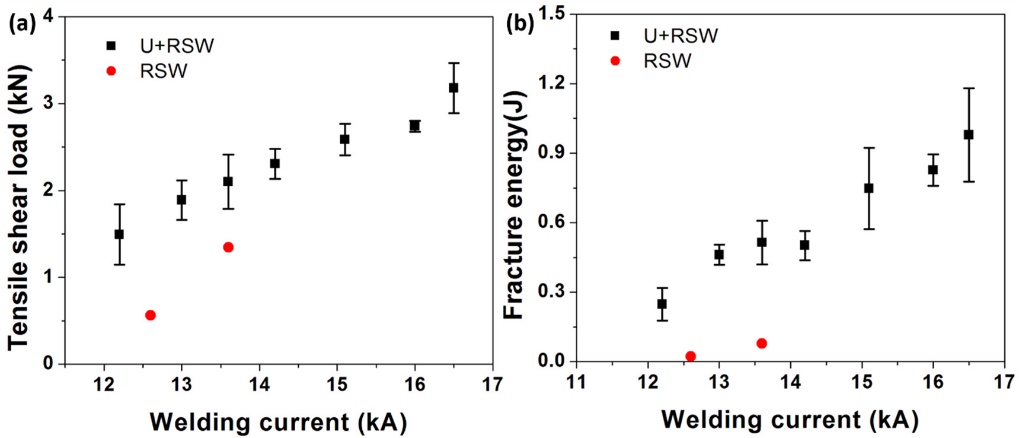
Figure 19. Effect of welding current on tensile shear strength and fracture energy of welded dissimilar joints of AA6061 to AISI 1008 steel. The welding time and the electrode force for RSW were kept constant at 0.083 s and 3.56 kN, respectively.L-17
Another research group has developed ultrasonic resistance welding process (URW), that apply in situ ultrasonic waves during the resistance spot welding process. S-17 The schematics of the process is shown in the Figure 20. Al-6061T6 and electrogalvanized TRIP-780 with dimensions of 70Lx25Wx1.6T mm3 and 70Lx25Wx1.3T mm3 respectively were used in this study.
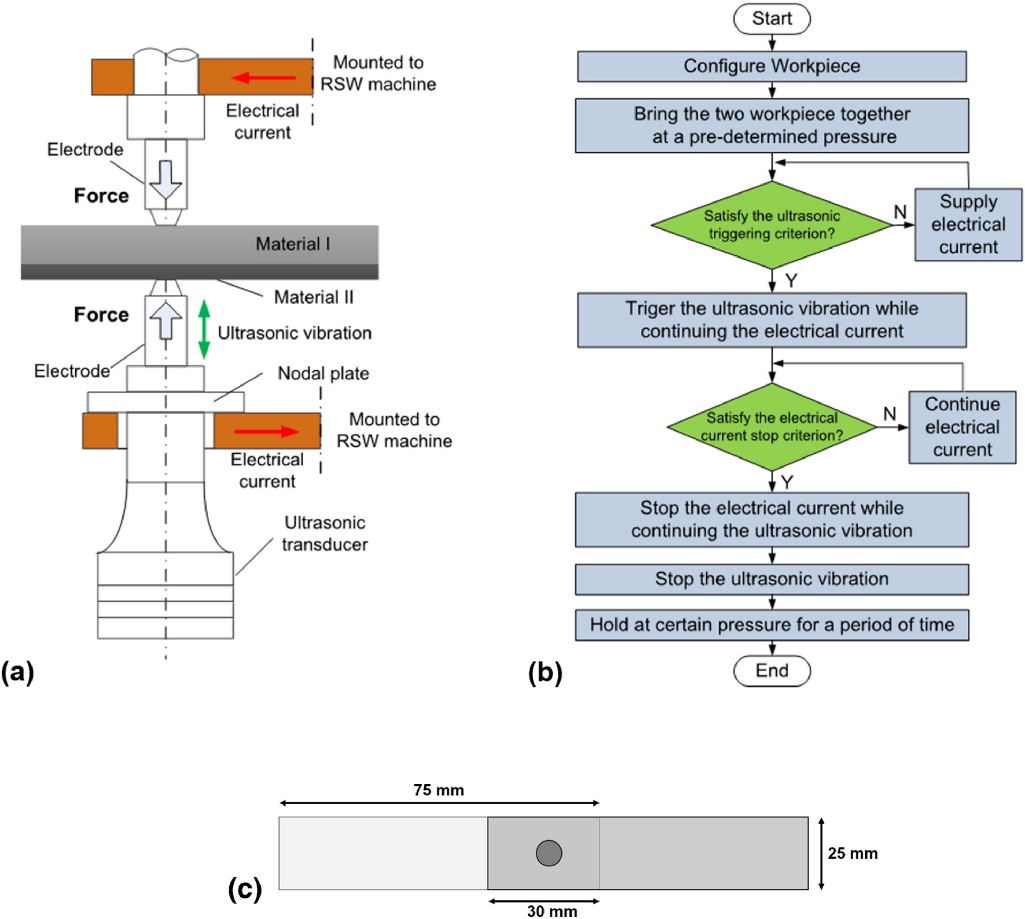
Figure 20. URW Setup (a) Schematic illustration; (b) Flowchart of the URW process.S-17
URW process have shown improved mechanical properties and defect free interface compared to RSW process. In situ ultrasonic vibration facilitated the breakdown of the surface oxide and contaminants, which modifies contact resistance and heat generation rate accordingly. If melting occurs, ultrasonic vibration promoted wetting of the molten aluminum over the steel interface and resulted in the formation defect free fully bonded interface as shown in Figure 22 compares the mechanical properties between the RSW & URW process. Comparing URW with traditional RSW, up to 300% increase in strength and more than 150% increase in displacement to failure is reported. SEM images of the fractured surface revealed that ultrasonic waves eliminated the eggcrate morphology generally observed in RSW welds fractured surface, which is a typical representative of solidification cracking.
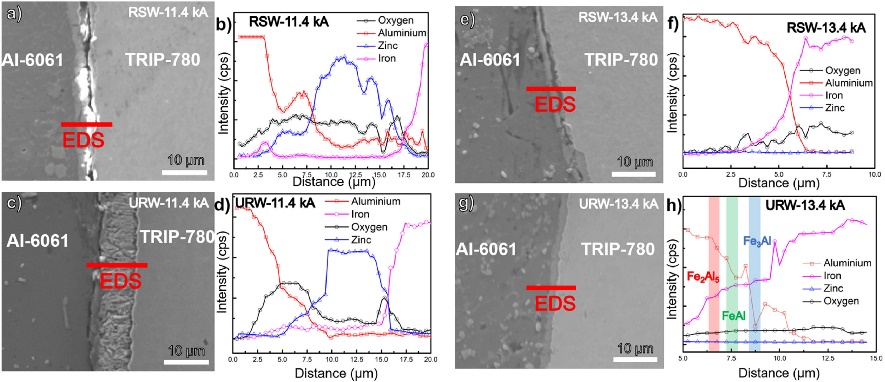
Figure 21. EDS line Scan of Al-6061 & TRIP 780 across interface for RSW at 11.4 kA (a-b) & at 13.4 kA (e-f) and for URW at 11.4 kA (c-d) & at 13.4 kA (g-h).S-17
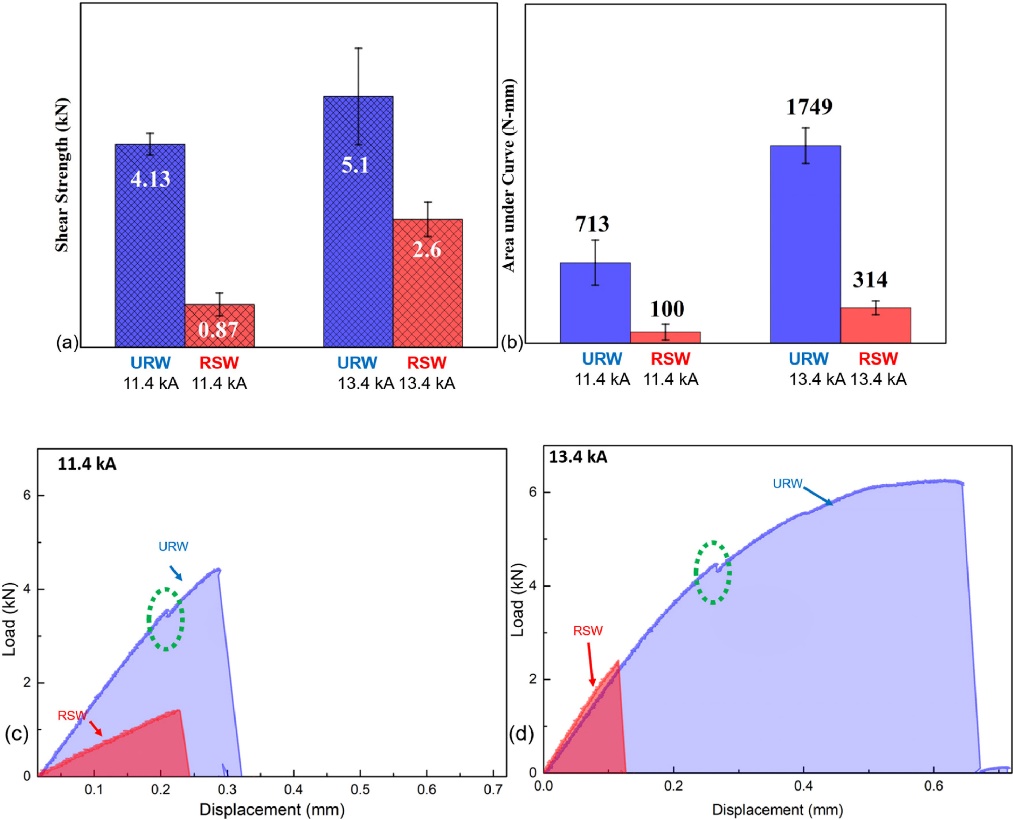
Figure 22: Tensile test results (a) Joint shear strength from 11.4 kA (30 cycles) & 13.4 kA (20 cycles); (b) Energy absorption calculated via area under load-displacement curve for 11.4 kA (30 cycles) & 13.4 kA (20 cycles); (c-d) load-displacement curve for 11.4.S-17
Back To Top
Solid State Welding
Introduction
Solid-State welding refers to a family of processes that produce welds without the requirement for molten metal. Solid-state welding theory emphasizes that the driving force for two pieces of metal to spontaneously weld (or form a metallic bond) to each other exists if the barriers (oxides, contaminants, and surface roughness) to welding can be eliminated. All solid-state welding processes are based on this concept, and use some combination of heat, pressure, and time to overcome the barriers. Approaches include friction, diffusion, explosion, and ultrasonic welding.
Since there is no melting, there is no chance of forming defects such as porosity or slag inclusions which are only associated with fusion welding processes. Solid-state welding processes also require no filler materials, and in some cases, can be quite effective at welding dissimilar metals that cannot be welded with conventional processes due to metallurgical incompatibilities. The equipment is typically very expensive, and some processes involve significant preparation time of the parts to be welded. Most of these processes are limited to certain joint designs, and some of them are not conducive to a production environment. Non Destructive Testing processes do not always work well with solid-state welding processes because of the difficulties of distinguishing a true metallurgical bond with these techniques.
In this section you’ll find examples of three types of solid state welding processes: Friction, High-Frequency for tubes and pipe and Magnetic Pulse. Generally these are characterized as follows. Click to the specific menu for details.
Friction Welding Processes
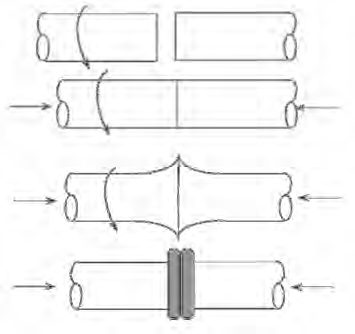
Figure 1: Basic steps of both inertia and CD friction welding.
This family of processes relies on significant plastic deformation or forging action to overcome the barriers to solid-state welding. Frictional heating dominates at the beginning of the process, followed by the heating due to plastic deformation once the forging action begins. The friction welding processes which use rotation of one part against another are inertia and continuous (or direct)-drive friction welding. These are the most common of the friction welding processes and are ideal for round bars or tubes. In both inertia and Continuous-Drive (CD) friction welding, one part is rotated at high speeds relative to the other part (Figure 1). They are then brought together under force creating frictional heating which softens (reduces the YS) the material at and near the joint to facilitate the forging action, which, in turn, produces further heating. Following a sufficient amount of time to properly heat the parts, a high upset force is applied which squeeze the softened hot metal out into the “flash”. Any contaminants are squeezed out as well as the weld is formed. The flash is usually removed immediately after welding while it is still hot.A-11, P-6
High Frequency Tube/Pipe Welding
HF welding processes rely on the properties of HF electricity and thermal conduction, which determine the distribution of heat in the workpieces. HF contact welding and high-frequency induction welding are used to weld products made from coil, flat, or tubular stock with a constant joint symmetry throughout the length of the weld.
Magnetic Pulse Welding (MPW)
MPW is a solid-state process that uses electromagnetic pressure to accelerate one workpiece to produce an impact against another workpiece. The metallic bond created by this process is similar to the bond created by explosion welding. MPW, also known as electromagnetic pulse or magnetic impact welding, is highly regarded for the capability of joining dissimilar materials.