Testing and Characterization
The Hole Expansion test (HET) quantifies the edge stretching capability of a sheet metal grade having a specific edge condition. Many variables affect hole expansion performance. By understanding the microstructural basis for this performance, steelmakers have been able to create new grades with better edge stretch capability.
Multiphase microstructures with large hardness differences between the phases, specifically islands of the very hard martensite surrounded by a softer ferrite matrix, may crack along the ferrite-martensite interface (Figure 1). The larger the size of the initiated damage site (due to edge shearing), the smaller the critical stress required for crack propagation.M-6 The microstructure and damage are key components of the Shear Affected Zone, or SAZ.

Figure 1: Features and mechanisms of damage initiation and propagation in Dual Phase steel.M-6
One metallurgical approach to improve sheared edge stretchability is targeting a homogeneous microstructure. Steel suppliers have engineered product offerings like complex phase steel, where extensive grain refinement (reducing the size of the ferrite and martensite grains) is achieved. Consequently, the size of the initial damage resulting from shearing is reduced, raising the critical stress for crack propagation to higher levels and reducing the likelihood for crack propagation. Additionally, reducing the difference in hardness between the soft ferrite phase and the hard martensite phase improves the hole expansion ratio. Changes in chemistry, hot rolling conditions and intercritical annealing temperatures are some of the methods used to achieve this. Such metallurgical trends can include a single phase of bainite or multiple phases including bainite and removal of large particles of martensite. This trend is shown in Figure 2, adapted from Citation M-11.
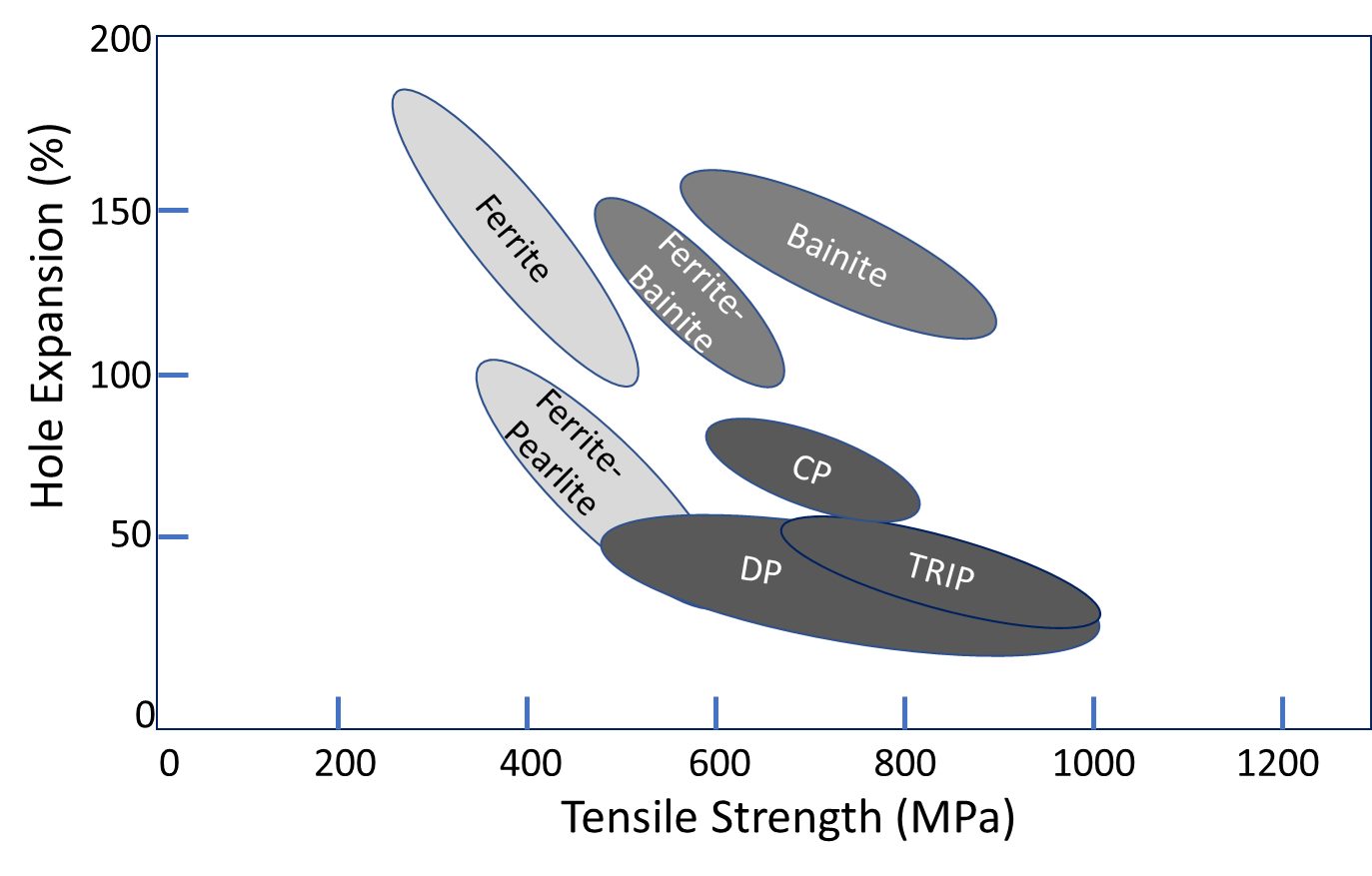
Figure 2: Hole Expansion as a Function of Strength and Microstructure. Adapted from Citation M-11.
An example of the impact of these modifications is shown in a paper published by C. Chiriac and D. HoydickC-10, where a 1 mm DP780 galvannealed steel was modified to produce a grade with improved hole expansion to achieve greater resistance to local formability failures such as edge fracture and shear fracture. These changes were made while retaining the same base metal chemistry and the same fraction of martensite in the structure, and resulted in similar tensile strength and total elongation but with a 50% increase in hole expansion (Table I and Figure 3). The key difference is a lower martensite hardness, and a smaller difference between the hardness of the martensite and ferrite. The modified DP grade has more homogeneous distribution of martensite with smaller ferrite and martensite grains (Figure 4).

Table I: Comparison of a conventional DP780 steel with a similar chemistry modified to improve hole expansion.C-10
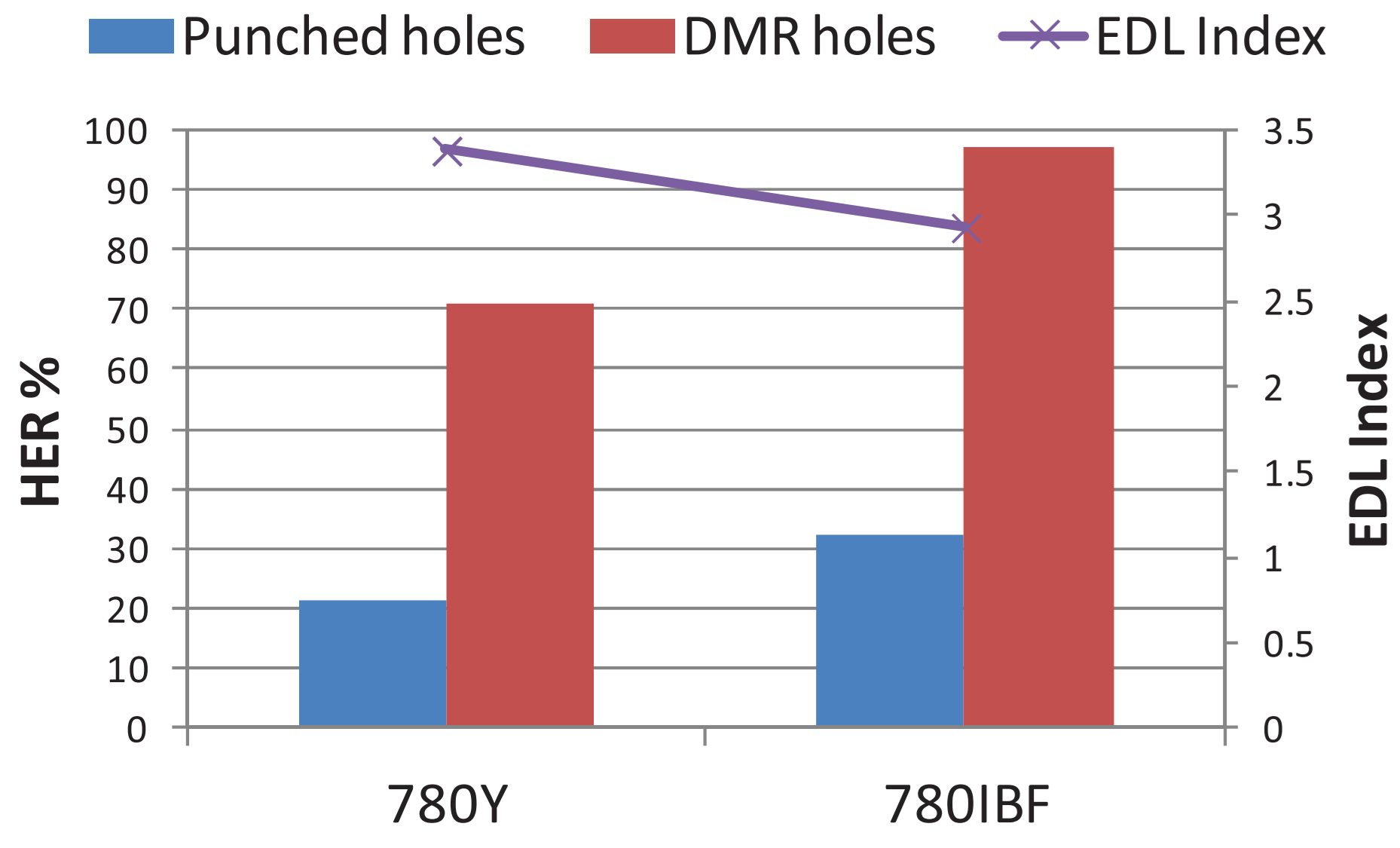
Figure 3: Improvement in Hole Expansion improves with grade modifications and edge quality. DMR = drilled, milled, and reamed hole; EDL = Edge Ductility Loss index, the ratio of the hole expansion of the DMR hole to that of the punched hole.C-10
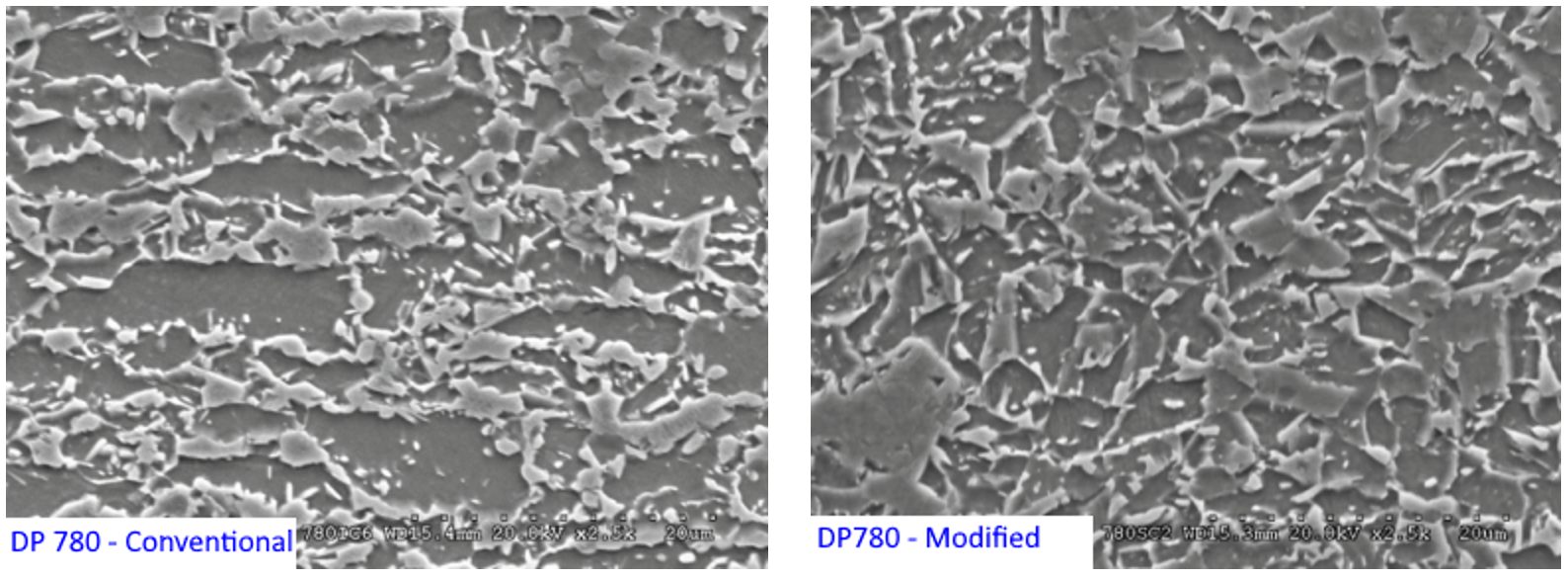
Figure 4: Comparison of the microstructure of a conventional DP780 steel (left) with a similar chemistry modified to improve hole expansion (right). Overall, there is the same fraction of martensite in both grades, but the modified chemistry has finer features.C-10
A presentation at a 2020 conferenceK-16 described a study which compared DP780 from six different global suppliers. Hole expansion tests were done on 1.4 mm to 1.5 mm mm thick samples prepared with either a sheared edge at 13% clearance, a sheared edge with 20% clearance, or a machined edge. Not surprisingly, the machined edge with minimal work hardening outperformed either of the sheared edge conditions. However, when considering only the machined edge samples, the hole expansion ratio ranged from below 30% to more than 70% (Figure 5). Presumably the only difference was the microstructural characteristics of the six DP780 products.

Figure 5: Variation in hole expansion performance from DP780 from 6 global suppliers.K-56
The microstructural differences that enhance local formability characteristics may be detrimental to global formability characteristics and vice versa. Conventional dual phase steels, with a soft ferrite matrix surrounding hard martensite islands, excel in applications where global formability is the limiting scenario. These steels have a low YS/TS ratio and high total elongation. However, the interface between the ferrite and martensite is the site of failures that limit the sheared edge extension of these grades. On the other end of the spectrum, fully martensitic grades are the highest strength steels available. These have a high YS/TS ratio, and low total elongation. Having only a single phase helps these grades achieve surprisingly high hole expansion values considering the strength, as seen in Figure 6.
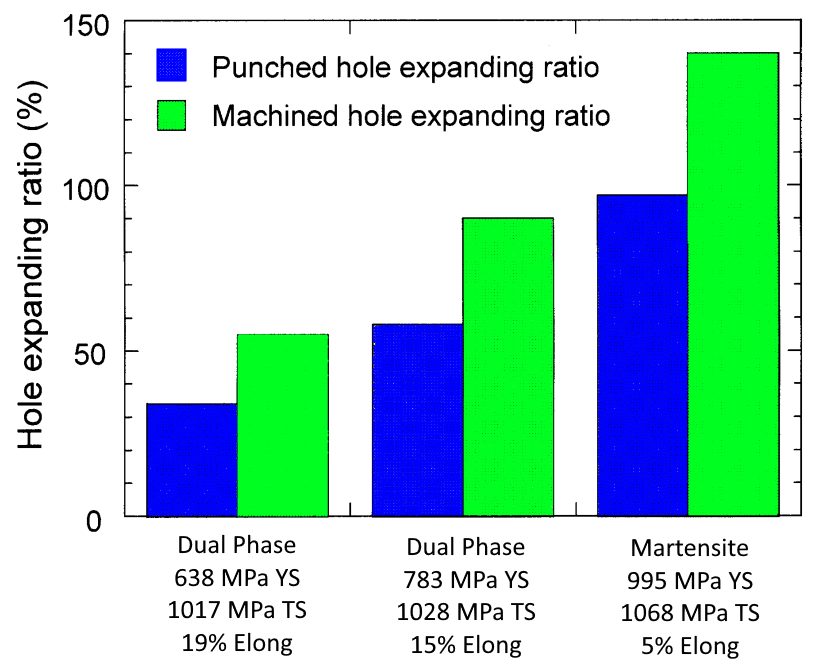
Figure 6: Hole Expansion as a Function of Edge Quality and Microstructure. Adapted from Citation.H-7
Knowing that a higher volume fraction of martensite is needed to increase strength, combined with the awareness that minimizing the hardness differences between microstructural phases is needed to increase hole expansion (Figure 7), allows steelmakers to fine-tune their chemistry and mill processing to target specific balances of strength, tensile elongation, and cut edge expandability as measured in a tensile test.
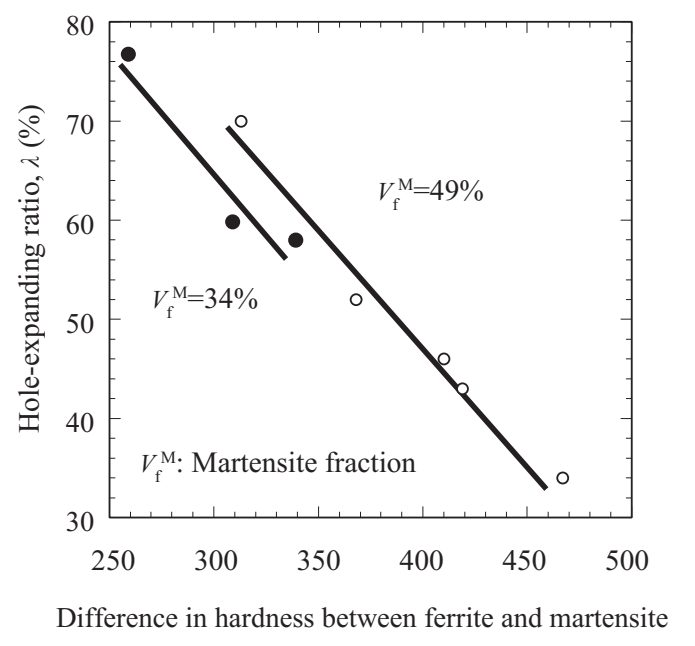
Figure 7: Improved Hole Expansion by Reducing the Hardness Difference between Ferrite and Martensite.H-8
This expands the selection of grades from which manufacturers can choose. Traditional material selection and identification may have been based on tensile strength to satisfy structural requirements – DP980 is a dual phase steel with 980MPa minimum tensile strength. However, newly engineered grade options offer users an extra level of refinement depending on the functional needs of the part. Products can be specified as needing high tensile elongation, high hole expansion, or a balance of these two. In the example shown in Figure 8, note that all 3 grades have nearly identical tensile strength.
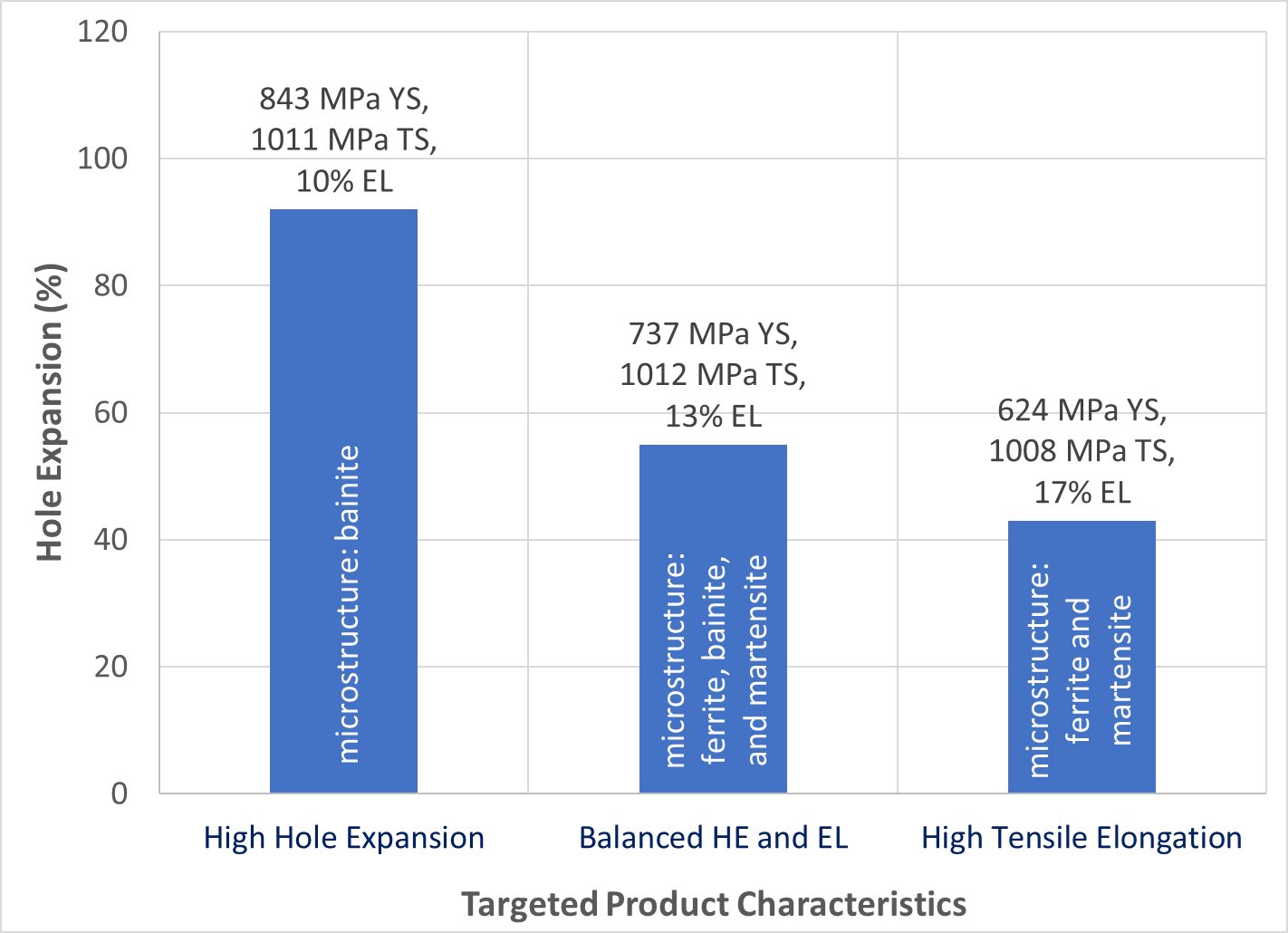
Figure 8: Engineered Microstructures Achieve Targeted Product Characteristics. (Data from Citations N-8 and F-5)
The influence of microstructure and the hardness differences between the phases is also seen in the hole expansion values of AHSS grades at strengths below 980 MPa. A study from 2016 shows the impact of a small amount of martensite on a ferrite-bainite microstructure.N-9 Both products compared had a microstructure of 80% ferrite. In one product, the remaining phase was only bainite, while the other had both martensite and bainite. The presence of just 8% martensite was sufficient to decrease the hole expansion capacity by 40%. (Figure 9).
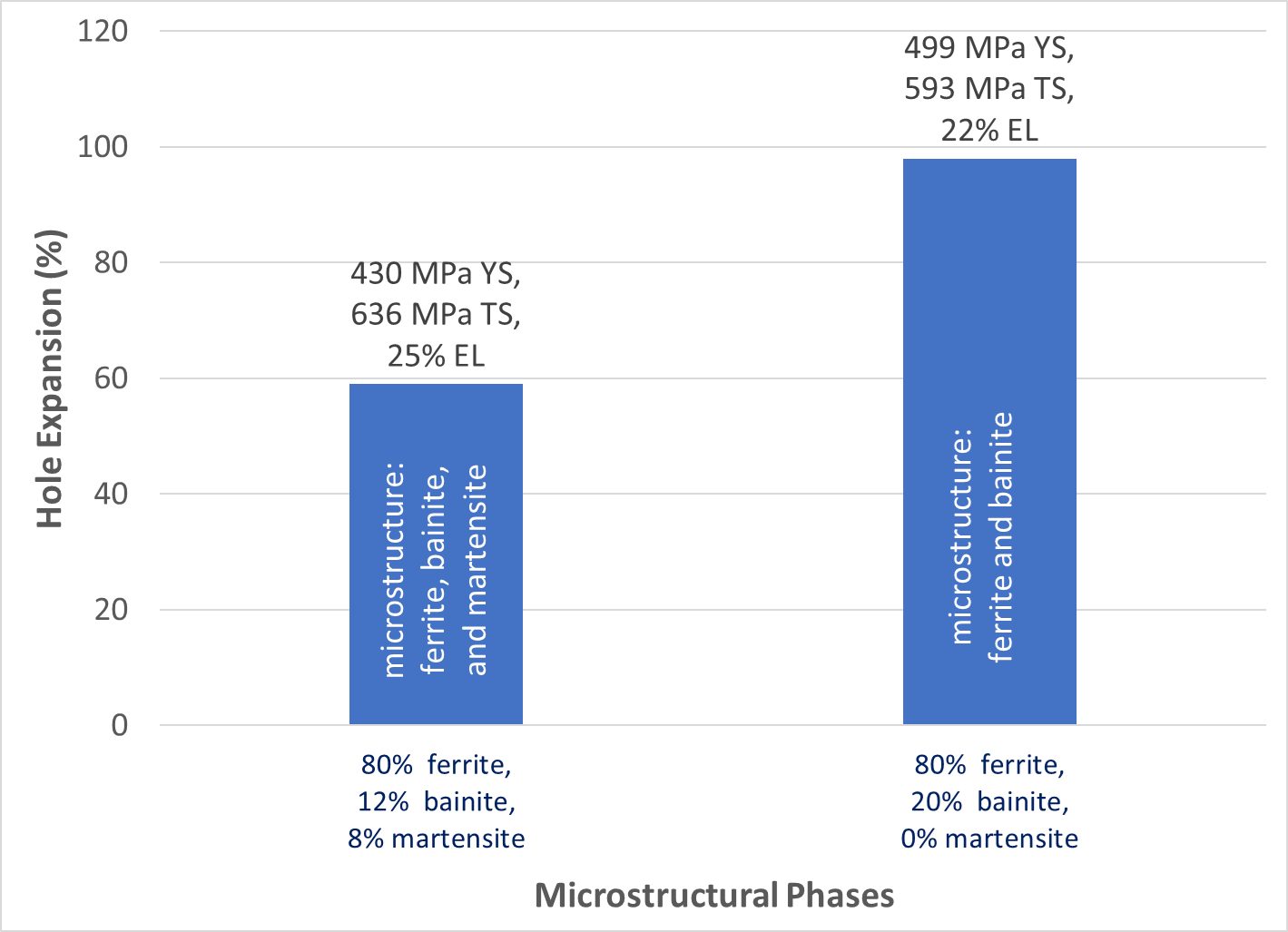
Figure 9: High Hardness Differences in Microstructural Phases Decrease Edge Ductility at All Strength Levels. Adapted from Citation N-9.
Rolling direction may also influence edge fracture sensitivity on some multiphase AHSS grades. When testing a sample, edge fractures may occur first at the hole edge along the rolling direction, which corresponds to a tensile axis in the transverse direction. If the chosen grade exhibits this behavior, locate stretch flanges perpendicular to the rolling direction when possible during die and process development to increase resistance to edge fracture. If this is not practical, identify locations where inserting scallops/notches in the stretch flange will not negatively impact the part structure, fit or die processing.
During die development and die try-out, it is important to use the production-intent AHSS grade – not just one that has the same tensile strength. Blank orientation relative to the rolling direction in these trials must also be production-intent. Often the blank die is the last completed die, so prototype blanks may be prepared by laser, EDM, water jet or even by hand during tryout. These cutting methods will have different sheared edge extension, as measured by the hole expansion test, compared with the production-intent shearing. These differences may be sufficiently significant to prevent replication of production conditions in tryout.
Cutting, Cutting-Blanking-Shearing-Trimming
Advanced High-Strength Steels (AHSS) exhibit high degrees of work hardening, resulting in improved forming capabilities compared to conventional HSLA steels. However, the same high work hardening creates higher strength and hardness in sheared or punched edges, leading to reduced edge ductility. Microstructural features in some AHSS grades contribute to their sheared edge performance. While laser cutting results in less edge damage than mechanical cutting methods, the heat from laser cutting produces a localized hear treatment, changing the strength and hardness at the edge. Achieving the best formability for chosen processing path requires generating a consistent good quality edge from the cutting operation.
To avoid unexpected problems during a program launch, use production intent tooling as early in the development as possible. This may be a challenge since blanking dies are usually among the last set of tools completed. In the interim, many companies choose to use laser cut blanks. Tool, blank, and process development must account for the lower-ductility sheared edges in production blanks.
Edge Ductility Measurements
This article describes the impact of cutting and cut-edge quality on edge ductility. The primary tests which quantify edge ductility are Hole Expansion Testing, 2-D Edge Tension Testing, and Half Specimen Dome Testing. These links detail the testing procedures. The Hole Expansion Testing article has additional information pertaining to the effect of burr orientation and punch shape.
Cut Edge Quality
Any mechanical cutting operation such as blanking, piercing, shearing, slitting, or trimming reduces edge ductility. Each of these processes generate a zone of high work hardening and a reduced n-value. This work hardened zone can extend one-half metal thickness from the cut edge. This is one reason why edges fail at strains lower than that predicted by the forming limit curve for that particular grade (Note that FLCs were developed based on necking failure, and that edge cracking is a different failure mechanism).
DP and TRIP steels have islands of martensite located throughout the ferritic microstructure, including at the cut edges. These hard particles act as crack initiators and further reduce the allowable edge stretch. Metallurgical changes to the alloy minimize the hardness differences between the phases, resulting in improved edge ductility. Laser, EDM or water jet cutting approaches minimize work hardening at the edges and the associated n-value reduction, also leading to improved edge ductility.
Putting shear angles into cutting tools is a well-known approach to reduce cutting forces. Modifying the cutting tool leads to other benefits in terms of edge ductility. Researchers studied the effects of a beveled punch instead of the traditional flat bottom punch.S-9, S-50, S-52 In these studies, the optimized bevel angle was between 3 and 6 degrees, the shear direction was parallel the rolling direction of the coil with a die clearance of 17%. With the optimal cutting parameters, the hole expansion ratio increased by 60% when compared to conventional flat punching process. As expected, a reduction in the maximum shearing force occurred – by more than 50% in certain conditions. Dropping the shearing force helps reduce the snap through reverse tonnage, leading to longer tool and press life.
Multiple studies examine the trimmed edge quality based on various cutting conditions in mechanical shearing operations and other methods to produce a free edge such as milling and cutting using a laser or water jet. Edge quality varies based on parameters like cutting clearances, shear angles, and rake angles on mechanical shearing operations.
A typical mechanically sheared steel edge has 4 main zones – rollover, burnish, fracture, and burr, as shown in Figure 1.

Figure 1: Cross Section of a Punched Hole Showing the Shear Face Components and Shear Affected Zone.K-10
Parts stamped from conventional mild and HSLA steels have historically relied on burr height as the main measure of edge quality, where the typical practice targeted a burr height below 10% of metal thickness and slightly larger for thicker steel. Finding a burr exceeded this threshold usually led to sharpening or replacing the trim steels, or less likely, adjusting the clearances to minimize the burr.
Greater burr height is associated with additional cold working and creates stress risers that can lead to edge splitting. These splits, however, are global formability related failures where the steel thins significantly at and around the split, independent of the local formability edge fractures associated with AHSS. A real-world example is shown in Figure 2, which presents a conventional BH210 steel grade liftgate with an excessive burr in the blank that led to global formability edge splitting in the draw die. The left image in Figure 2 highlights the burr on the underside of the top blank, with the remainder of the lift below it. The areas next to the split in the right image of Figure 2 shows the characteristic thinning associated with global formability failures.
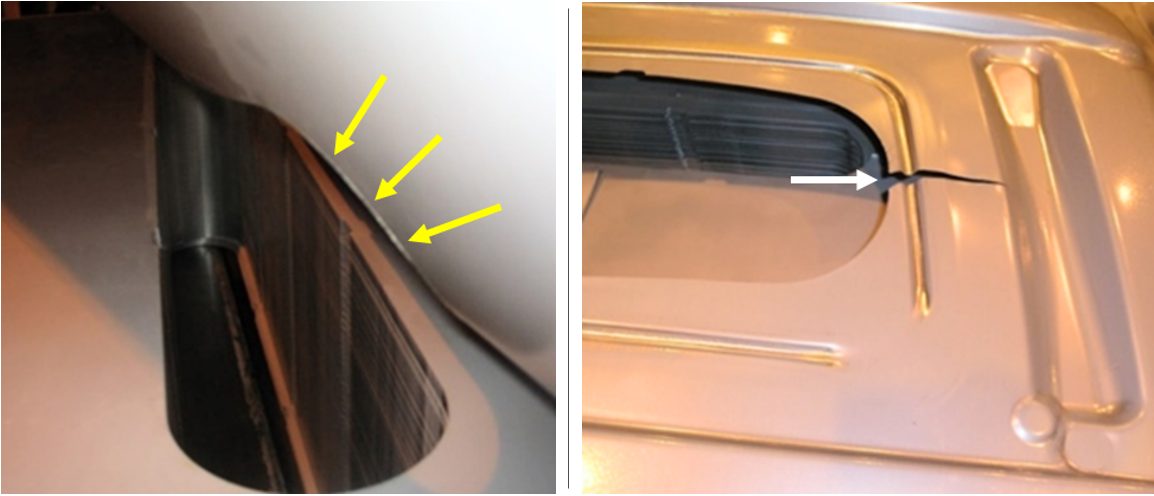
Figure 2: Excessive burr on the blank led to a global formability split on the formed liftgate. The root cause was determined to be dull trim steels resulting in excessive work hardening.U-6
Due to their progressively higher yield and tensile strengths, AHSS grades experience less rollover and smaller burrs. They tend to fracture with little rollover or burr. As such, detailed examination of the actual edge condition under various cutting conditions becomes more significant with AHSS as opposed characterizing edge quality by burr height alone. Examination of sheared edges produced under various trimming conditions, including microhardness testing to evaluate work hardening after cold working the sheared edge, provides insight on methods to improve cut edge formability. The ideal condition to combat local formability edge fractures for AHSS was to have a clearly defined burnish zone with a uniform transition to the fracture zone. The fracture zone should also be smooth with no voids, secondary shear or edge damage (Figure 3).

Figure 3: Ideal sheared edge with a distinct burnish zone and a smooth fracture zone (left) and a cross section of the same edge (right).U-6
If clearances are too small, secondary shear can occur and the potential for voids due to the multiphase microstructure increases, as indicated in Figure 4. Clearances that are too large create additional problems that include excessive burrs and voids. A nonuniform transition from the burnish zone to the fracture zone is also undesirable. These non-ideal conditions create propagation sites for edge fractures.

Figure 4: Sheared edge with the trim steel clearance too small (left) and a cross section of the same edge (right) showing a micro crack on the edge. Tight clearance leading to secondary shear increases likelihood of edge fracture.U-6
There are multiple causes for a poor sheared edge condition, including but not limited to:
- the die clearance being too large or too small,
- a cutting angle that is too small,
- worn, chipped, or damaged tooling,
- improperly ground or sharpened tooling,
- improper die material,
- improperly heat-treated die material,
- improper (or non-existent) coating on the tooling,
- misaligned die sections,
- worn wear plates, and
- out of level presses or slitting equipment.
The higher loads required to shear AHSS with increasingly higher tensile strength creates additional deflection of dies and processing equipment. This deflection may alter clearances measured under a static condition once the die, press, or slitting equipment is placed under load. As a large percentage of presses, levelers, straighteners, blankers, and slitting equipment were designed years ago, the significantly higher loads required to process today’s AHSS may exceed equipment beyond their design limits, dramatically altering their performance.
A rocker panel formed from DP980 provides a good example showing the influence of cut edge quality. A master coil was slit into several narrower coils (mults) before being shipped to the stamper. Only a few mults experienced edge fractures, which all occurred along the slit edge. Understanding that edge condition is critical with respect to multiphase AHSS, the edge condition of the “good” mults and the “bad” mults were examined under magnification. The slit edge from a problem-free lift (Figure 5) has a uniform burnish zone with a uniform transition to the smooth fracture zone. This is in contrast with Figure 6, from the slit edge from a different mult of the same coil in which every blank fractured at the slit edge during forming. This edge exhibits secondary shear as well as a thick burnish zone with a non-uniform transition from the burnish zone to the fracture zone.

Figure 5: Slit edges on a lift of blanks that successfully produced DP980 rocker panels. Note the uniform transition from the burnish zone to the fracture zone with a smooth fracture zone as well.U-6

Figure 6: Slit edges on a lift of blanks from the same master coil that experienced edge fractures during forming. Note the obvious secondary shear as well as the thicker, nonuniform transition from the burnish to the fracture zone.U-6
Cutting Clearances: Burr Height and Tool Wear
Cutting and punching clearances should be increased with increasing sheet material strength. The clearances range from about 6% of the sheet material thickness for mild steel up to 16% or even higher as the sheet metal tensile strength exceeds 1400 MPa.
A study C-2 compared the tool wear and burr height formation associated with punching mild steel and several AHSS grades. In addition to 1.0 mm mild steel (140 MPa yield strength, 270 MPa tensile strength, 38% A80 elongation), AHSS grades tested were 1.0 mm samples of DP 350Y600T (A80=20%), DP 500Y800T (A80=8%), and MS 1150Y1400T (A80 = 3%). Tests of mild steel used a 6% clearance and W.Nr. 1.2363 / AISI A2 tool steel hardened to 61 HRC. The AHSS tests used engineered tool steels made from powder metallurgy hardened to 60-62 HRC. The DP 350/600 tests were run with a TiC CVD coating, and a 6% clearance. Tool clearances were 10% for the MS 1150Y1400T grade and 14% for DP 500Y800T.
In the Tool Wear comparison, the cross-section of the worn punch was measured after 200,000 hits. Punches used with mild steel lost about 2000 μm2 after 200,000 hits, and is shown in Figure 7 normalized to 1. The relative tool wear of the other AHSS grades are also shown, indicating that using surface treated high quality tool steels results in the same level of wear associated with mild steels punched with conventional tools.

Figure 7: Tool wear associated with punching up to DP 500Y800T using surface treated high quality tool steels is comparable to mild steel punched with conventional tools.C-2
Figure 8 shows the burr height test results, which compared burr height from tests using mild steel punched with conventional tool steel and two AHSS grades (DP 500Y800T and MS 1150Y1400T) punched with a PM tool steel. The measured burr height from all AHSS and clearance combinations evaluated were sufficiently similar that they are shown as a single curve.

Figure 8: Burr height comparison for mild steel and two AHSS grades as a function of the number of hits. Results for DP 500Y800T and Mart 1150Y1400T are identical and shown as the AHSS curve.C-2
Testing of mild steel resulted in the expected performance where burr height increases continuously with tool wear and clearance, making burr height a reasonable indicator of when to sharpen punching or cutting tools. However, for the AHSS grades studied, burr height did not increase with more hits. It is possible that the relatively lower ductility AHSS grades are not capable of reaching greater burr height due to fracturing, where the more formable mild steel continues to generate ever-increasing burr height with more hits and increasing tool wear.
Punching AHSS grades may require a higher-grade tool steel, possibly with a surface treatment, to avoid tool wear, but tool regrinding because of burrs may be less of a problem. With AHSS, engineered tool steels may provide longer intervals between sharpening, but increasing burr height alone should not be the only criterion to initiate sharpening: cut edge quality as shown in the above figures appears to be a better indicator. Note that regrinding a surface treated tool steel removes the surface treatment. Be sure to re-treat the tool to achieve targeted performance.
Cutting Clearances: General Recommendations
Depending on the source, the recommended die clearance when shearing mild steels is 5% to 10% of metal thickness. For punched holes, these represent per-side values. Although this may have been satisfactory for mild steels, the clearance should increase as the tensile strength of the sheet metal increases.
The choice of clearance impacts other aspects of the cutting process. Small cutting clearances require improved press and die alignment, greater punching forces, and cause greater punch wear from abrasion. As clearance increases, tool wear decreases, but rollover on the cut edge face increases, which in the extreme may lead to a tensile fracture in the rollover zone (Figure 9). Also, a large die clearance when punching high strength materials with a small difference in yield and tensile strength (like martensitic grades) may generate high bending stresses on the punch edge, which increases the risk of chipping.

Figure 9: Large rollover may lead to tensile fracture in the rollover zone.
Figure 10 compares cut edge appearance after punching a martensitic steel with 1400 MPa tensile strength using either 6% or 14% clearance. The larger clearance is associated with greater rollover, but a cleaner cut face.

Figure 10: Cut edge appearance after punching CR 1400T-MS with 6% (left) and 14% (right) die clearance. The bottom images show the edge appearance for the full sheet thickness, Note using 6% clearance resulted in minimal rollover, but uneven burnish and fracture surfaces. In contrast, 14% clearance led to noticeable rollover, but a clean burnish and fracture surface.T-20
A comparison of the edges of a 2 mm thick complex phase steel with 700 MPa minimum tensile strength produced under different cutting conditions is presented in Figure 11. The left image suggests that either the cutting clearance and/or the shearing angle was too large. The right image shows an optimal edge likely to result in good edge ductility.

Figure 11: Cut edge appearance of 2 mm HR 700Y-MC, a complex phase steel. The edge on the right is more likely to result in good edge ductility.T-20
The recommended clearance is a function of the sheet grade, thickness, and tensile strength. Figures 12 to 15 represent general recommendations from several sources.

Figure 12: Recommended Clearance as a Function of Grade and Sheet Thickness.T-23

Figure 13: Recommended Cutting Clearance for Punching.D-15

Figure 14: Recommended die clearance for blanking/punching advanced high strength steel.T-20

Figure 15: Multiply the clearance on the left with the scaling factor in the right to reach the recommended die clearance.D-16
Figure 16 highlights the effect of cutting clearance on CP1200, and reinforces that the historical rule-of-thumb guidance of 10% clearance does not apply for all grades. In this studyU-3, increasing the clearance from 10% to 15% led to a significant improvement in hole expansion. The HER resulting from a 20% clearance was substantially better than that from a 10% clearance, but not as good as achieved with a 15% clearance. These differences will not be captured when testing only to the requirements of ISO 16630, which specifies the use of 12% clearance.

Figure 16: Effect of hole punching clearance on hole expansion of Complex Phase steel grade CP1200.U-3
Cutting speed influences the cut edge quality, so it also influences the optimal clearance for a given grade. In a study published in 2020G-49, higher speeds resulted in better sheared edge ductility for all parameters evaluated, with those edges having minimal rollover height, smoother sheared surface and negligible burr. Two grades were evaluated: a dual phase steel with 780MPa minimum tensile strength and a 3rd Generation steel with 980 MPa minimum tensile strength.
Metallurgical characteristics of the sheet steel grade also affects hole expansion capabilities. Figure 17 compares the HER of DP780 from six global suppliers. Of course, the machined edge shows the highest HER due to the minimally work-hardened edge. Holes formed with 13% clearance produced greater hole expansion ratios than those formed with 20% clearance, but the magnitude of the improvement was not consistent between the different suppliers.K-56

Figure 17: Cutting clearance affects hole expansion performance in DP780 from six global suppliers.K-56
Punch Face Design
Practitioners in the field typically do not cut perpendicular to the sheet surface – angled punches and blades are known to reduce cutting forces. For example, long shear blades might have a 2 to 3 degree angle on them to minimize peak tonnages. There are additional benefits to altering the punch profile and impacting angle.
Snap-though or reverse tonnage results in stresses which may damage tooling, dies, and presses. Tools may crack from fatigue. Perhaps counter to conventional thinking, use of a coated punch increases blanking and punching forces. The coating leads to lower friction between the punch and the sheet surface, which makes crack initiation more difficult without using higher forces.
Unlike a coated tool, a chamfered punch surface reduces blanking and punching forces. Figure 18 compares the forces to punch a 5 mm diameter hole in 1 mm thick MS-1400T using different punch shapes. A chamfered punch was the most effective in reducing both the punching force requirements and the snap-through tonnage (the shock waves and negative tonnage readings in Figure 18). The chamfer should be large enough to initiate the cut before the entire punch face is in contact with the sheet surface. A larger chamfer increases the risk of plastic deformation of the punch tip.T-20

Figure 18: A chamfered punch reduces peak loads and snap-through tonnage.K-15
A different study P-16 showed more dramatic benefits. Use of a rooftop punch resulted in up to an 80% reduction in punching force requirements compared with a flat punch, with a significant reduction in snap-through tonnage. Cutting clearance had only minimal effect on the results. (Figure 19)

Figure 19: A rooftop-shaped punch leads to dramatic reductions in punch load requirements and snap-through tonnage.P-16
Use of a beveled punch (Figure 20) provides similar benefits. A study S-52 comparing DP 500/780 and DP 550/980 showed a reduction in the maximum piercing force of more than 50% with the use of a beveling angle between 3 and 6 degrees. The shearing force depends also upon the die clearance during punching, with the optimum performance seen with 17% die clearance. The optimal punching condition results in more than 60% improvement in the hole expansion ratio when compared to conventional flat head punching process. The optimal bevel cut edge in Figure 21 shows a uniform burnish zone with a uniform transition to the smooth fracture zone – the known conditions to produce a high-ductility edge.

Figure 20: Schematic showing a beveled punch.S-52

Figure 21: A bevel cut edge showing uniform burnish zone with a uniform transition to the smooth fracture zone.S-52
Effect of Edge Preparation Method on Ductility
A flat trim condition where the upper blade and lower blade motions are parallel and there is no shear rake angle is known to produce a trimmed edge with limited edge stretchability (Figure 22, left image). In addition to split parts, tooling damage and unexpected down time results. Metal stampers have known that shearing with a rake angle Figure 22, right image) will reduce cutting forces compared with using a flat cut. With advanced high strength steels, there is an accompanying reduction in forming energy requirements of up to 20% depending on the conditions, which represents a tremendous drop in snap-through or reverse tonnage. Figure 22 visually describes the upper and lower blade rake angles and the shear rake angle.
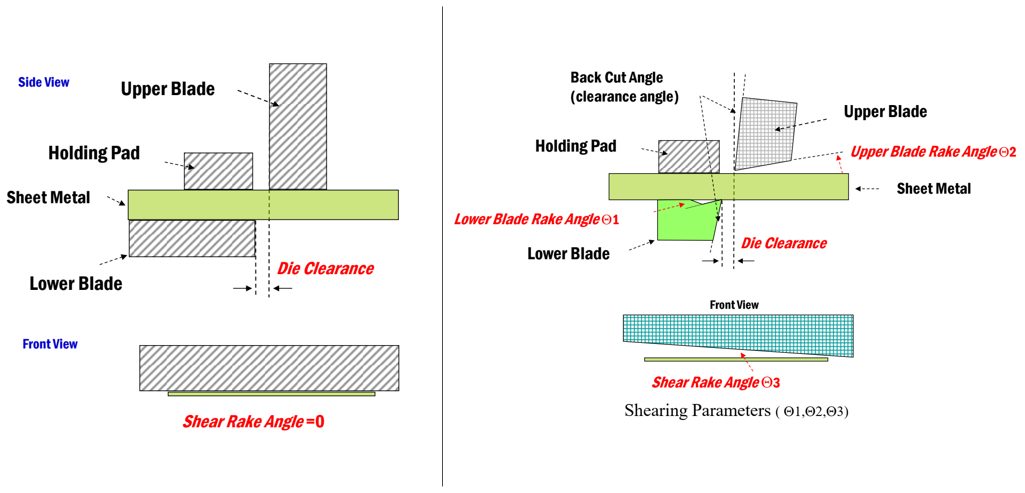
Figure 22: Flat trim (left) and shear trim (right) conditions showing rake angle definitions.S-53
Researchers have also found that it is possible to increase sheared edge ductility with optimized rake angles. Citation S-53 used 2-D Edge Tension Testing and the Half-Specimen Dome Test to qualify the effects of these rake angles, and determine the optimum settings. After preparing the trimmed edge with the targeted conditions, the samples were pulled in a tensile test or deformed using a hemispherical punch. The effect of the trimming conditions was seen in the measured elongation values and the strain at failure, respectively. The results are summarized in Figures 23-25. Some of the tests also evaluated milled, laser trimmed, and water jet cut samples. Shear Trim 1, 2, and 3 refer to the shear trim angle in degrees. The optimized shear condition also includes a 6-degree rake angle on both the upper and lower blades, as defined in Figure 22.
Conclusions from this study include:
- Mechanically shearing the edge cold works the steel and reduces the work hardening exponent (n-value), leading to less edge stretchability.
- Samples prepared with processes that avoided cold working the edges, like laser or water jet cutting outperformed mechanically sheared edges.
- Optimizing the trim shear conditions or polishing a flat trimmed edge approaches what can be achieved with laser trimming and water jet cutting.
- Shearing parameters such as clearance, shear angle and rake angle also play a large part in improving edge stretch.

Figure 23: Effect of edge preparation on stretchability as determined using a tensile test for DP 350Y600T (left) and DP 550Y980T (right).S-53

Figure 24: Effect of edge preparation on stretchability as determined using a dome test for DP 350Y600T (left) and DP 550Y980T (right).S-53

Figure 25: Optimizing the trim shear conditions or polishing a flat trimmed edge approaches what is achievable with laser trimming and water jet cutting. Data from dome testing of DP 350Y/600T.S-53
The optimal edge will have no mechanical damage and no microstructural changes as you go further from the edge. Any process that changes the edge quality from the bulk material can influence performance. This includes the mechanical damage from shearing operations, which cold works the edge leading to a reduction in ductility. Laser cutting also changes the edge microstructure, since the associated heat input is sufficient to alter the engineered balance of phases which give AHSS grades their unique properties. However, the heat from laser cutting is sometimes advantageous, such as in the creation of locally softened zones to improve cut edge ductility in some applications of press hardening steels.
The effects of edge preparation on the shear affected zone is presented in Figure 26. A flatter profile of the Vickers microhardness reading measured from the as-produced edge into the material indicates the least work-hardening and mechanical damage resulting from the edge preparation method, and therefore should result in the greatest edge ductility. This is certainly the case for water jet cutting, where a flat hardness profile in Figure 26 correlates with the highest ductility measurements in Figures 22 to 25. Unfortunately, water jet cutting is not always practical, and introduces the risk of rust forming at the newly cut edge.
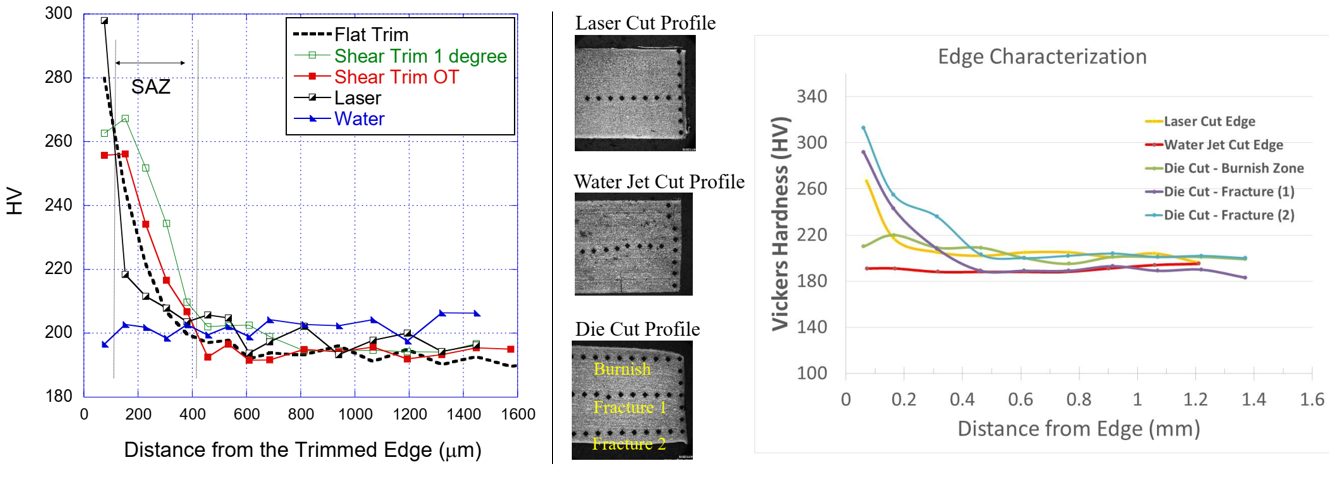
Figure 26: Microhardness profile starting at cut edge generated using different methods. Left image is from Citation S-53, and right image is from C-13.
Two-stage piercing is another method to reduce edge strain hardening effects. Here, a conventional piercing operation is followed by a shaving operation which removes the work-hardened material created in the first step, as illustrated in Figure 27.P-17 A related studyF-10 evaluated this method with a 4 mm thick complex phase steel with 800 MPa tensile strength. Using the configuration documented in this reference, single-stage shearing resulted in a hole expansion ratio of only 5%, where the addition of the shaving operation improved the hole expansion ratio to 40%.

Figure 27: Two-stage piercing improves cut edge ductility. Image adapted from Citation P-17.
Figure 28 highlights the benefits of two-stage pre-piercing for specific grades, showing a 2x to 4x improvement in hole expansion ratio for the grades presented.

Figure 28: Pre-piercing improves the hole expansion ratio of AHSS Grades.S-10
Key Points
- Clearances for punching, blanking, and shearing should increase as the strength of the material increases, but only up to a point. At the highest strengths, reducing clearance improves tool chipping risk.
- Lower punch/die clearances lead to accelerated tool wear. Higher punch/die clearances generate more rollover/burr.
- ISO 16630, the global specification for hole expansion testing, specifies the use of 12% punch-to-die clearance. Optimized clearance varies by grade, so additional testing may prove insightful.
- Recommended clearance as a percentage of sheet thickness increases with thickness, even at the same strength level.
- Burr height increases with tool wear and increasing die clearances for shearing mild steel, but AHSS tends to maintain a constant burr height. This means extended intervals between tool sharpening may be possible with AHSS parts, providing edge quality and edge performance remain acceptable.
- Edge preparation methods like milling, laser trimming, and water-jet cutting minimize cold working at the edges, resulting in the greatest edge ductility,
- Laser cut blanks used during early tool tryout may not represent normal blanking, shearing, and punching quality, resulting in edge ductility that will not occur in production. Using production-intent tooling as early as possible in the development stage minimizes this risk.
- Shear or bevel on punches and trim steel reduces punch forces, minimizes snap-through reverse tonnage, and improves edge ductility.
- Mild steel punched with conventional tools and AHSS grades punched with surface treated engineered PM tool steels experience comparable wear.
- Maintenance of key process variables, such as clearance and tool condition, is critical to achieving long-term edge stretchability.
- The optimal edge appearance shows a uniform burnish zone with a uniform transition to a smooth fracture zone.
Back To Top
Testing and Characterization
The term local formability describes when part and process design, in addition to sheet metal properties like strength and elongation, influence the amount of deformation the metal can undergo prior to failure. Cutting, punching or other methods of obtaining a trimmed blank or an internal hole results in cracks, rough edges, work-hardening and other edge damage – all of which influences edge quality. The challenges of capturing all of the factors that influence edge quality makes the prediction of fracture severity and cut edge expansion very difficult and usually impossible. The many variables highlight the need for a standardized test method. However, restricted sample preparation and testing variables in these standards do not reflect the variety of conditions encountered in production environments. Use caution when comparing results generated under different conditions.
Hole Expansion Testing
The Hole Expansion test (HET) quantifies the edge stretching capability of a sheet metal grade having a specific edge condition. Higher values of the hole expansion ratio are associated with grades and forming methods more likely to have improved local formability characteristics.
Steel producers study hole expansion capacity to create new products with targeted edge stretching performance through modifications of chemistry, rolling and thermal practices. Product designers use the hole expansion test to determine if the chosen steel grade has the inherent forming characteristics to meet their targeted shape with their chosen forming system. If they are not compatible, the chosen grade must change or aspects of the forming process must change, or possibly both.
ISO 16630 is the primary standard used which describes the test method and constraints.I-9 Others, like JIS Z 2256J-6 are based on the ISO standard, with only minor differences, if any. This standard specifies use of a 10mm diameter hole created with a 12% clearance. The sample containing the hole is clamped in place, and a conical punch having a 60 degree apex angle expands that initial hole (Figure 1). The test stops after observation of a through-thickness crack or upon experiencing a load-drop exceeding a critical threshold (Figure 2). The hole expansion ratio (HER), also known as the Hole Expansion Capacity (HEC), is simply the percent expansion of the diameter of the initial hole, typically shown as the Greek letter lambda, λ.
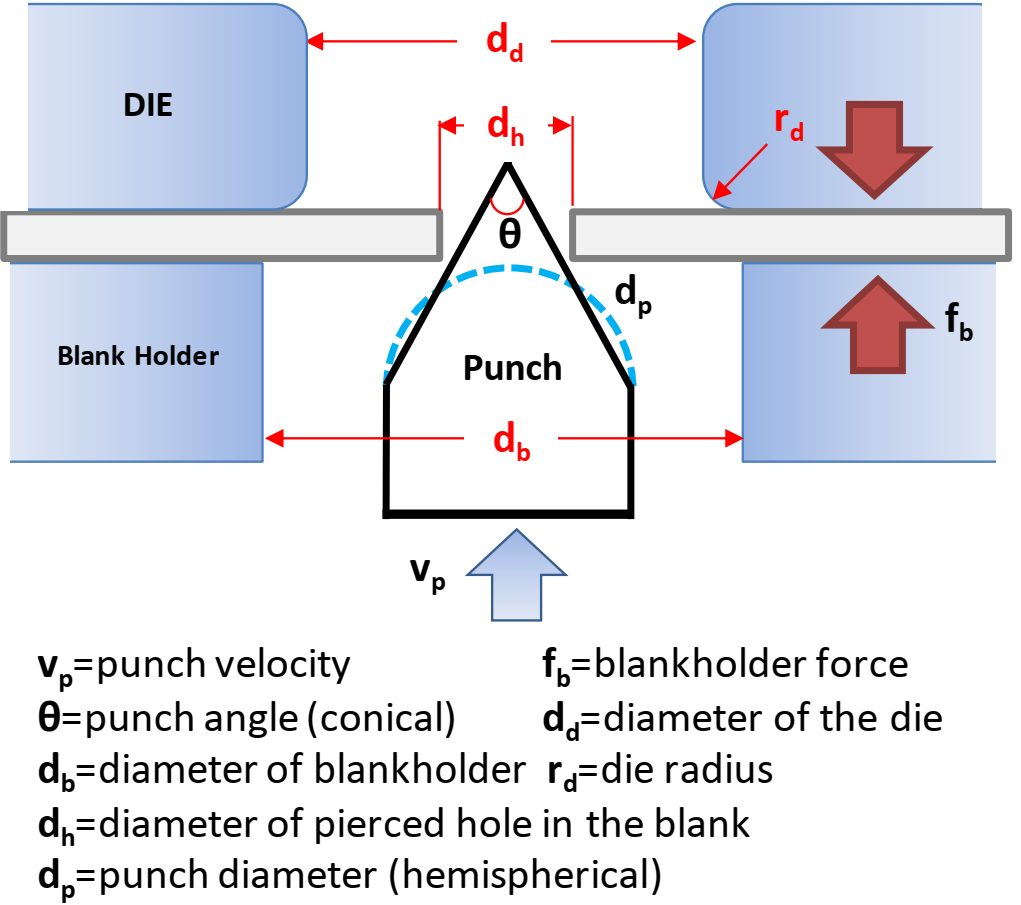
Figure 1: Schematic of Hole Expansion Test.A-10
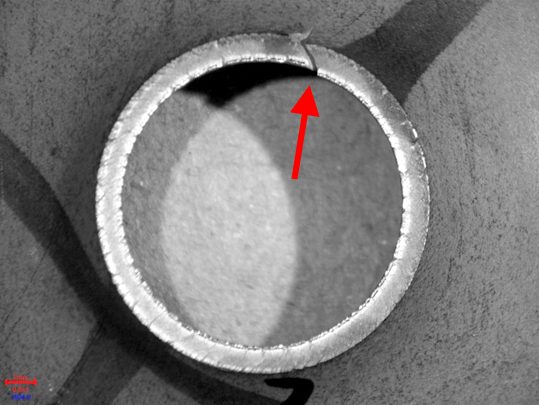
Figure 2: Expanded Edge at the end of a Hole Expansion Test performed using a conical punch. The arrow points to the through-thickness crack that ended the test.E-2
The sample preparation and testing requirements of ISO 16630 are well-defined for good reason. Factors known to influence the hole expansion ratio include:
Even with these rigorously defined procedures, the test results can be heavily influenced by specimen preparation technique, specific test parameters, and human subjectivity – in other words, poor gage R&R (repeatability and reproducibility). For example a group of European steel researchers reported “an unacceptably large difference between labs” with regard to hole expansion testing. They ultimately concluded that the “difference is too large for the method to be useful in practice”.
A-76
Testing sheet steels of different thicknesses in a laboratory setting requires having multiple punches and/or dies of different diameter to maintain a consistent clearance, which is based on a percentage of the sheet thickness tested.
In production, the punch-to-die clearance can change during the life of the part, both from tooling wear as well as press misalignment. There is the additional risk that clearance can vary around the perimeter of the cut section, leading to inconsistent performance. Increasing sheet metal strength magnifies this issue.
The method used to create the free edge influences the edge quality. Improved edge quality and reduced mechanical work hardening of the edge is achieved by laser cutting, EDM cutting, water jet cutting, or fine blanking processes, and will typically improve the hole expansion ratio. Trim steel clearances, shear angles, tool steel types, and sharpness also impact hole expansion test results.
In the example shown in Figure 3, the hole expansion ratio is reduced from 280% for a milled or water jet edge down to 80% for a traditional cut edge. If clearances further increase – which could happen without proper tooling maintenance over the life of the part – the ability to expand a cut edge further decreases.
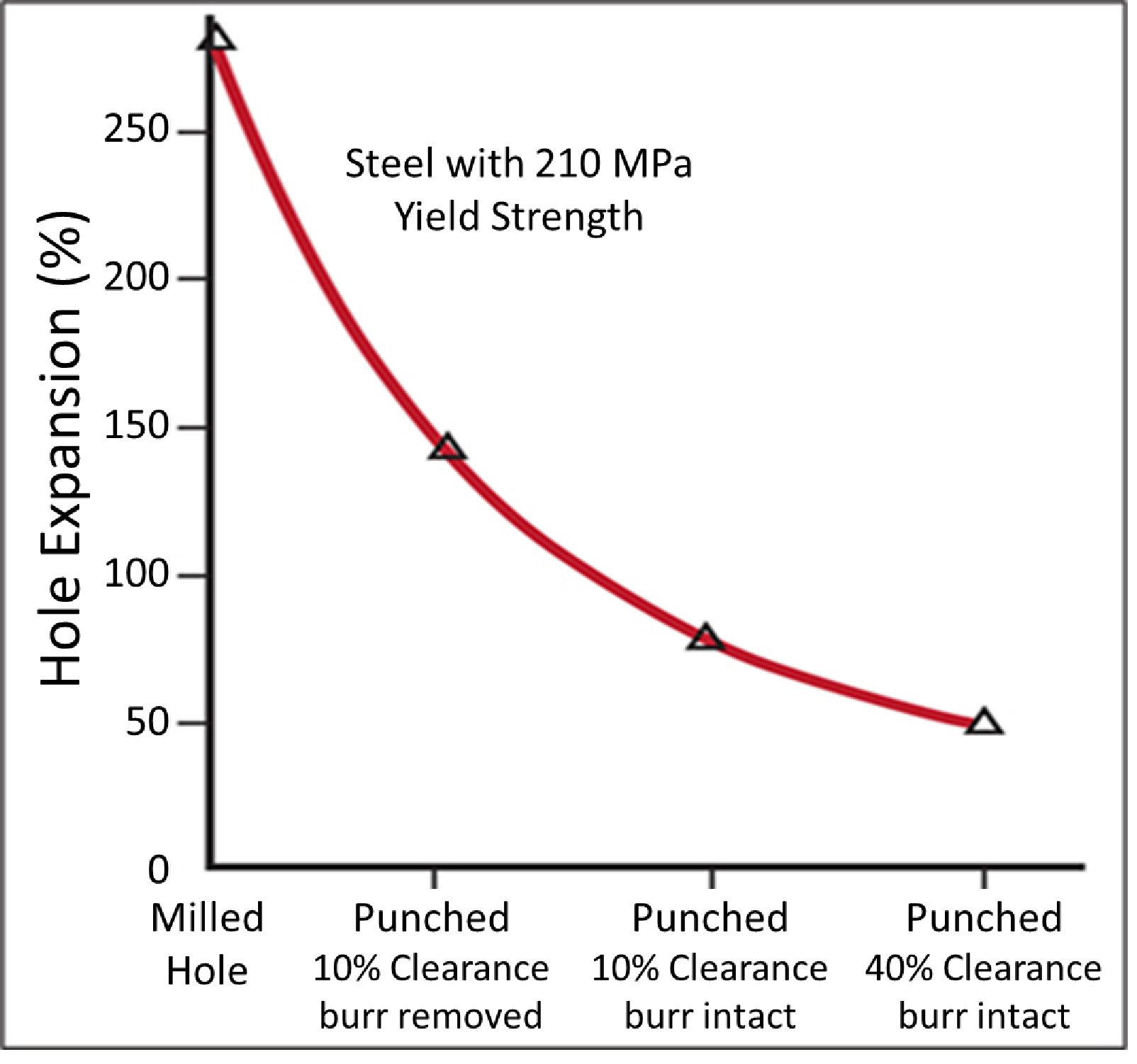
Figure 3: Hole Expansion Capacity Decreased as Edge Quality Decreases. (Based on data from Citation H-1.)
Figure 4 highlights the effect of punched vs machined holes, showing the edge damage from punching lowers the hole expansion capability. This edge damage becomes a key component of what is known as the Shear Affected Zone, or SAZ. DP steels and TRIP steels have a large hardness difference between the constituent phases, and therefore are associated with lower hole expansion ratios than HSLA and CP steels, where the phases are of more similar hardness. The influence of the metallurgical phase hardness difference is explored here. Detailed studies of sheared edge stretchability as a function of clearance, edge preparation, and grade are shown in Citations K-6 and K-10.
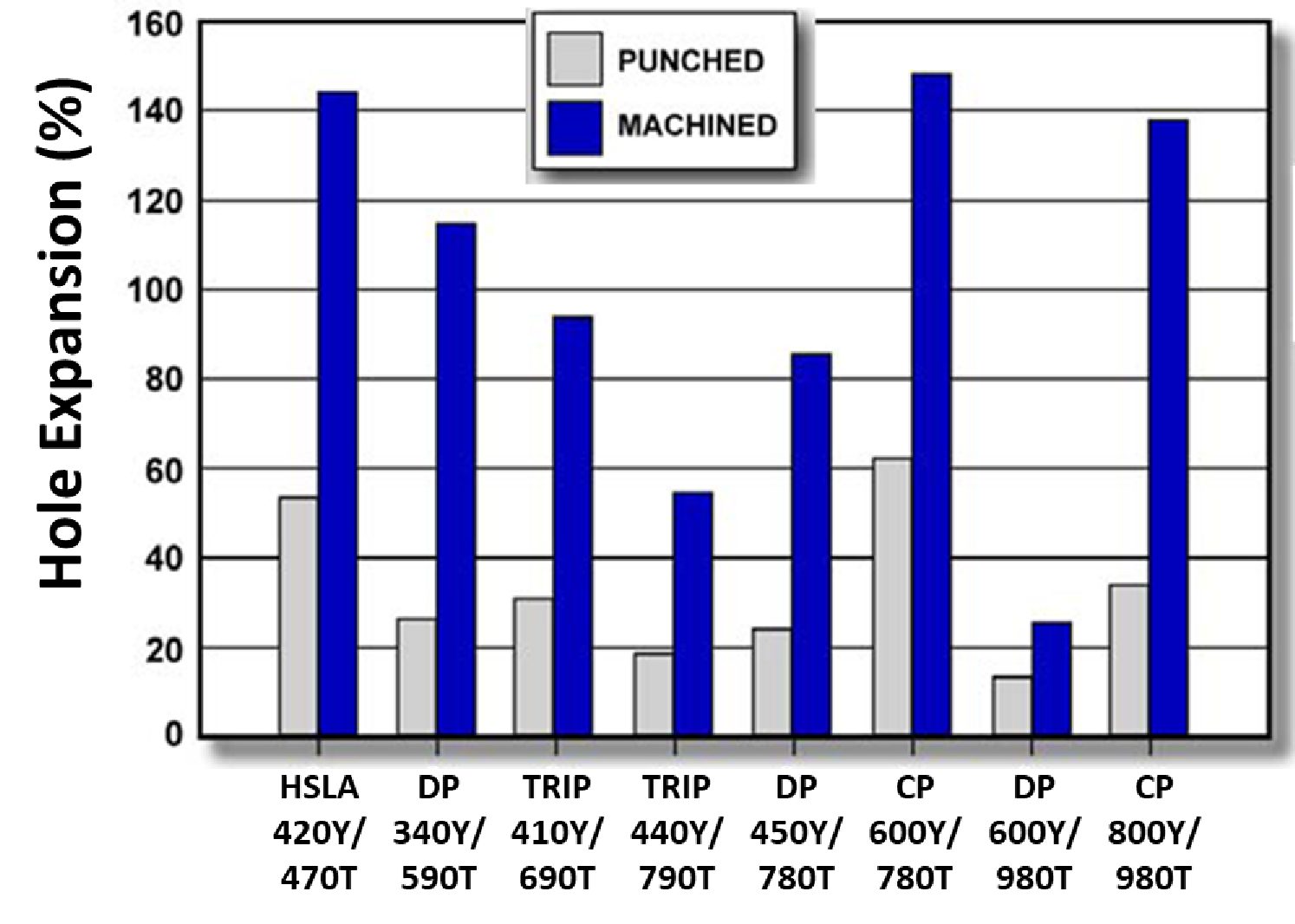
Figure 4: Hole expansion test results comparing punched and machined holes showing effect of damage to edge stretchability. (Based on data from Citation V-1.)
Over time, the targeted edge quality degrades and targeted clearance changes without proper attention. A study documented in Citation C-1 evaluated the hole expansion ratio created by hole punching tools as they wore in a production environment. Tools evaluated were made from 60 HRC uncoated Powder Metallurgy tool steels. Data in Figure 5 show the percent hole expansion from newly ground punches and dies (Sharp Tools) and from used production punches and dies (Worn Tools). The radial clearance was 0.1 mm. A rust preventative oil was applied to the steels during the punching; a lubricant oil was applied during hole expansion. Tool wear and possible micro-chipping resulted in a poor edge condition. The clearance was not significantly affected, but the steel edges suffered cold work which dramatically affected their hole expansion results.
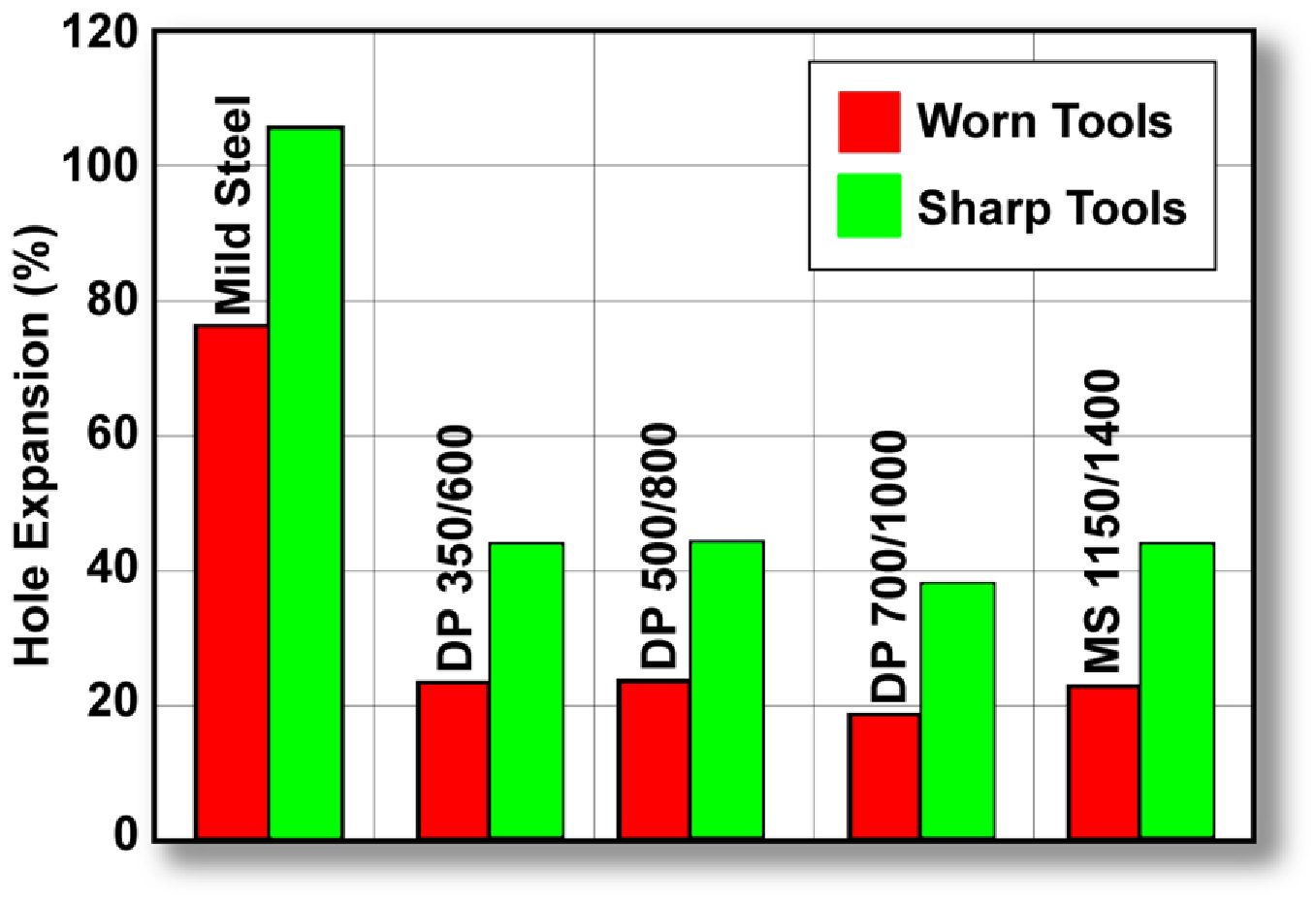
Figure 5: Impact of production tooling condition on hole expansion performance. (tests conducted w 50 mm diameter conical punch).C-1
Conclusions from Citation C-1 include:
- The best quality edge condition will produce the best results
- Tooling must remain sharp and damage-free to maintain the consistency in edge conditions.
- The burr should be in contact with the punch rather than on the freely-expanding side
- Hard and wear resistant tools, such as those produced from coated powder metallurgy (PM) tool steels, are highly recommended.
Additional information on tool materials can be found here and other articles in that category.
The ISO 16630 specificationI-9 eliminates one variable by prescribing the use of a 10 mm diameter hole, but it is important to understand that starting hole diameter influences the degree to which that hole can be expanded. A study that included mild steels to AHSS grades evaluated the effect of starting hole diameter.I-10 All steels were 1.2mm, punched with a clearance of 12.5%, and expanded with a conical punch having a 60° apex angle. As the starting diameter increases, the degree to which the hole can be expanded decreases, Figure 6. Note that as the strength increases, this effect appears to be minimized.
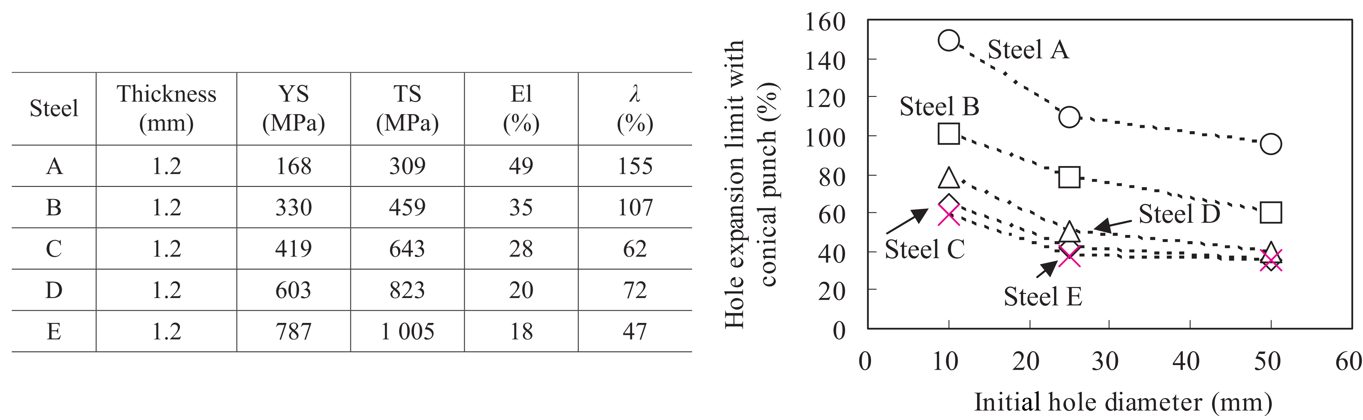
Figure 6: Hole Expansion Ratio Decreases as Initial Hole Diameter Increases.I-10
Increasing the starting hole diameter may help to distinguish between different grades.K-11 Similar hole expansion performance exists between DP980 and TRIP780 under ISO 16630 test conditions (punched 10 mm hole). It is easier to discern better performance in the TRIP780 product when performing a similar test with a 75 mm diameter punched hole (Figure 7).
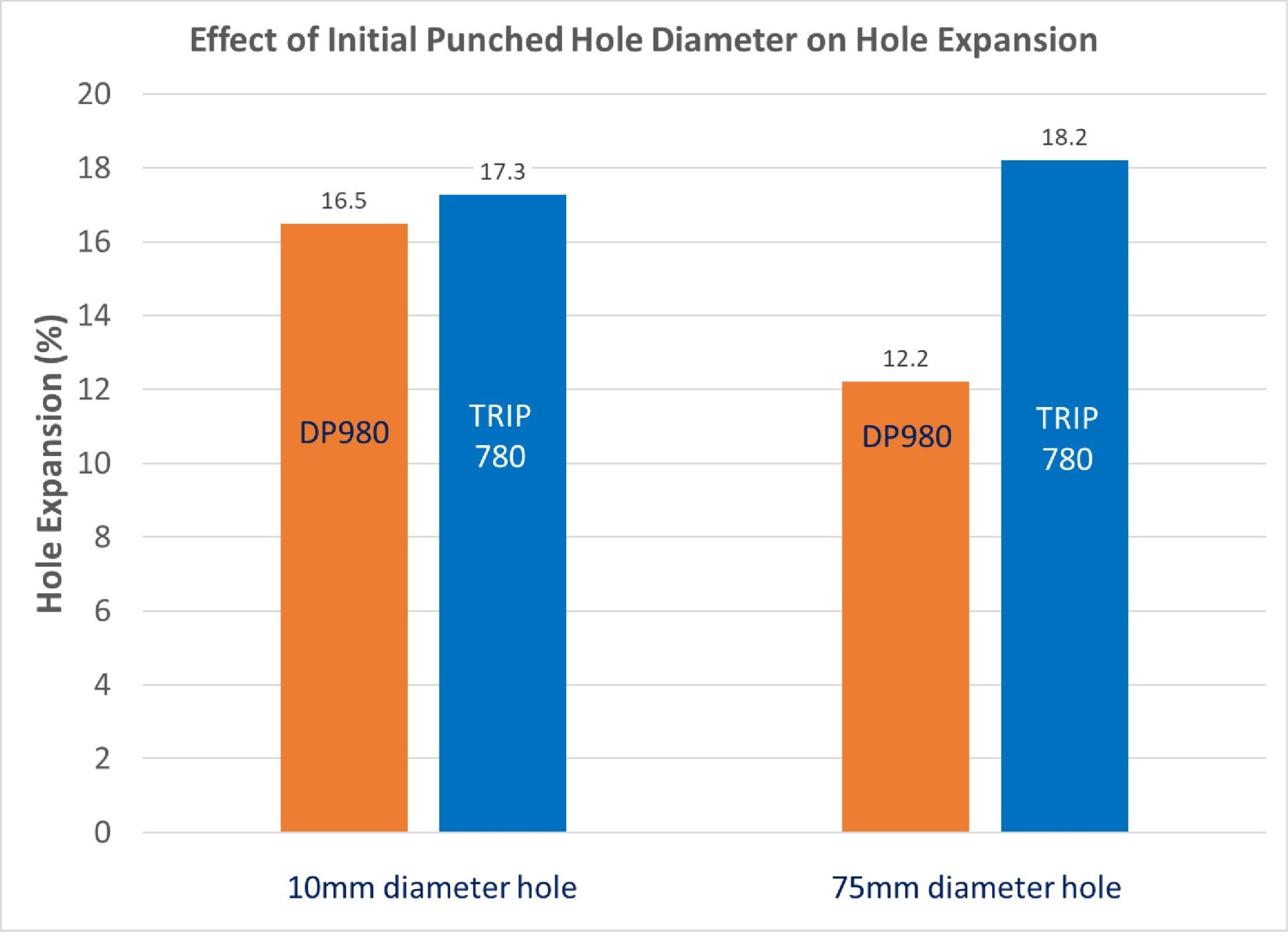
Figure 7: Effect of Initial Punched Hole Diameter on Hole Expansion. (Based on Data from Citation K-11.)
The position of the burr relative to the punch affects performance in a hole expansion test. Detrimental effects of an expanding edge are minimized If the burr is on the punch side. Having the burr on the punch side, rather than the freely expanding side, minimizes the detrimental effects of the expanding edge. The primary reason is the outer surface is in a greater degree of tension than the surface next to the punch.
Figure 8 examines the effect of edge condition and clearance on DP 590 expanded with a conical punch.K-10 The data suggests that there could be up to a 20% increase in sheared edge extension capability just related to the burr position on holes punched with conventional clearances. This should be considered in die processing materials and designs sensitive to edge expansion.
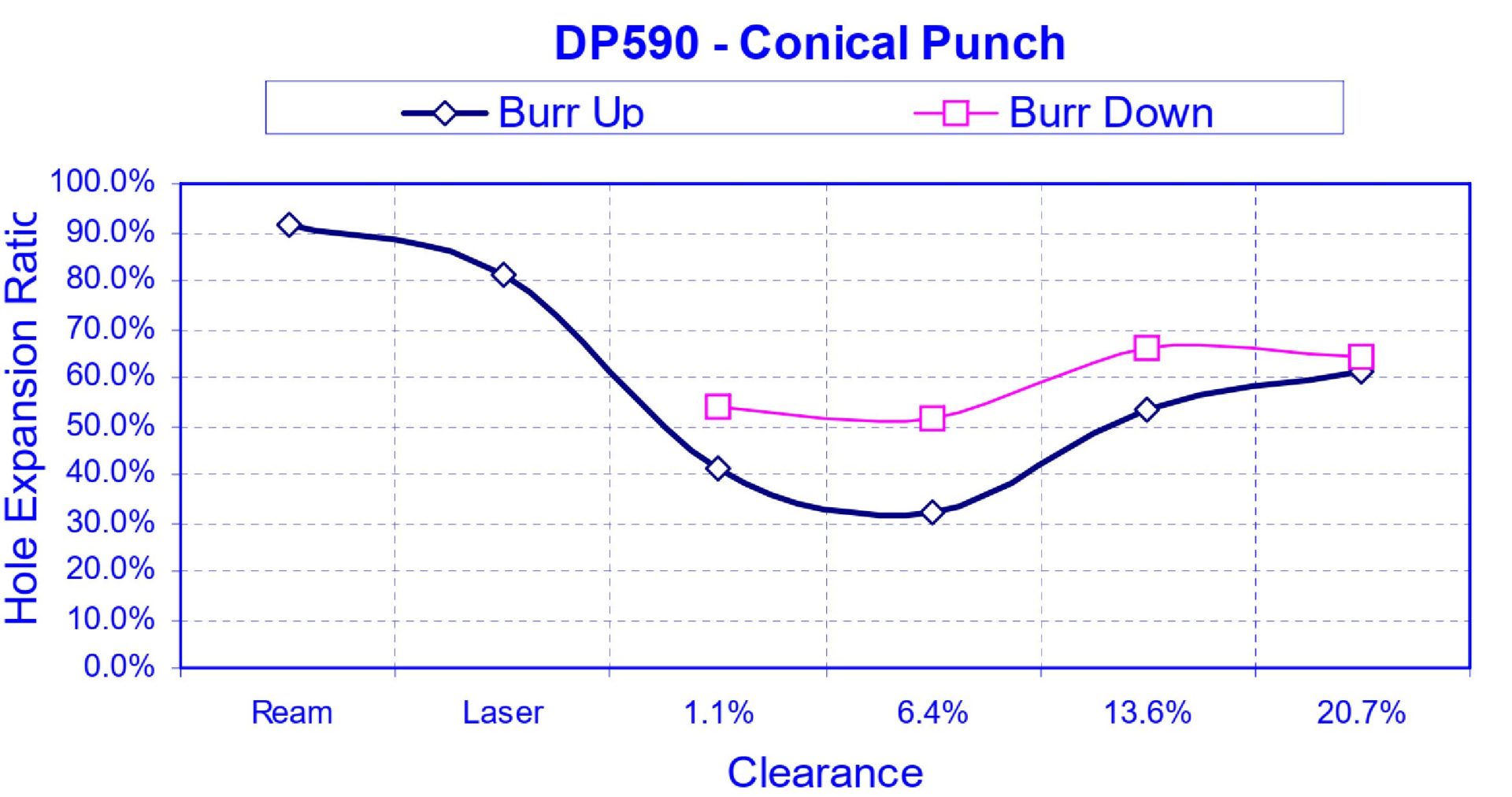
Figure 8: The Effect of Burr Orientation on Hole Expansion as a Function of Clearance on DP590. “Burr Up” means away from the punch; “Burr Down” means in contact with the punch.K-10
Shown in Figure 9 is the influence of burr orientation and material grade.K-10 The 50XK grade shown is HSLA 350Y/450T, where there is a significant improvement in the measured hole expansion related to the position of the burr relative to the punch. The magnitude of this difference decreases as strength increases, but persists for all grades tested.
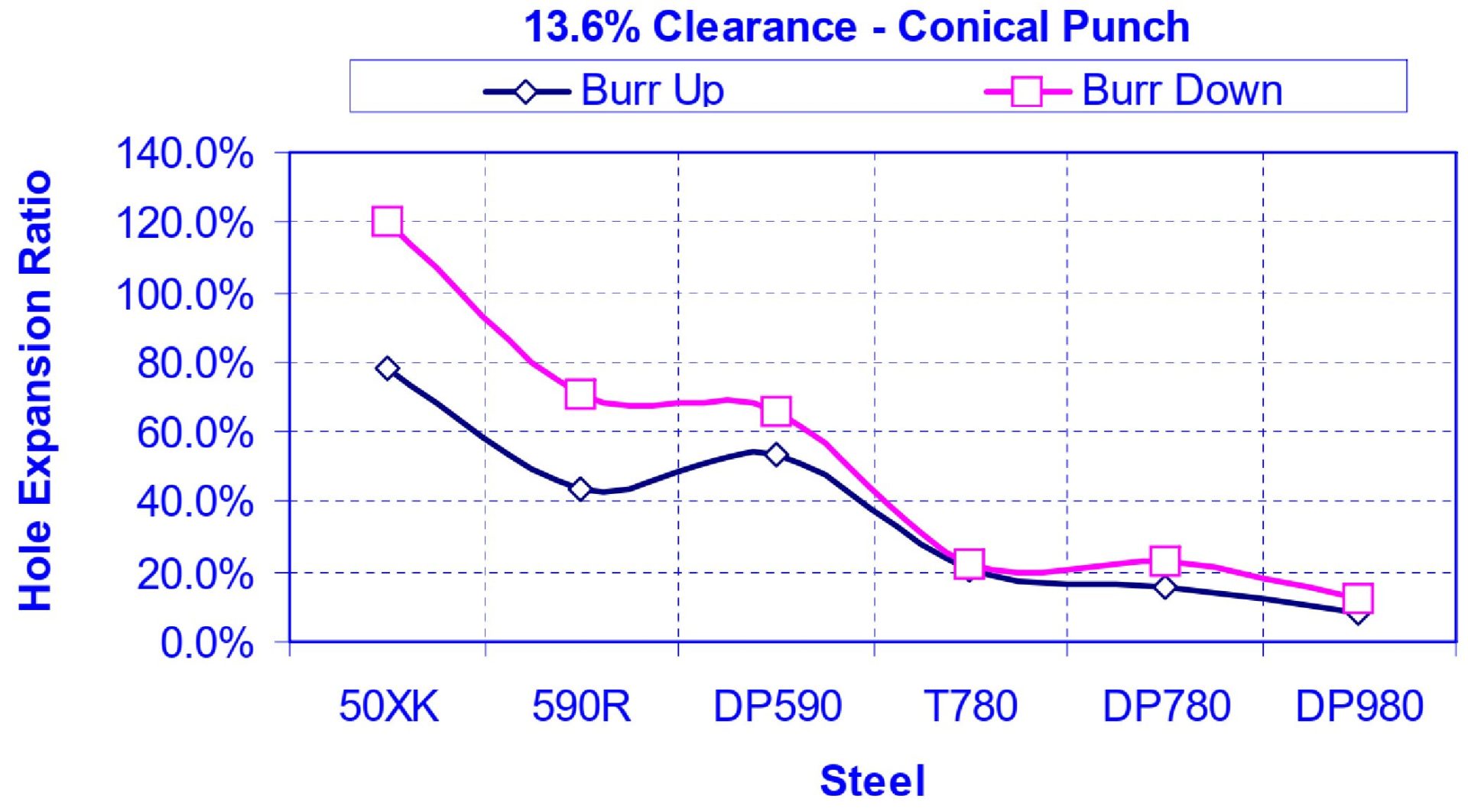
Figure 9: The Effect of Burr Orientation on Hole Expansion as a Function of Different High Strength Steel Grades “Burr Up” means away from the punch; “Burr Down” means in contact with the punch.K-10
The shape of the punch used to expand the hole impacts the degree to which it can be expanded. Figure 10 shows generalizations of the three most-common shapes: a conical punch, a flat punch, and a hemispherical punch.
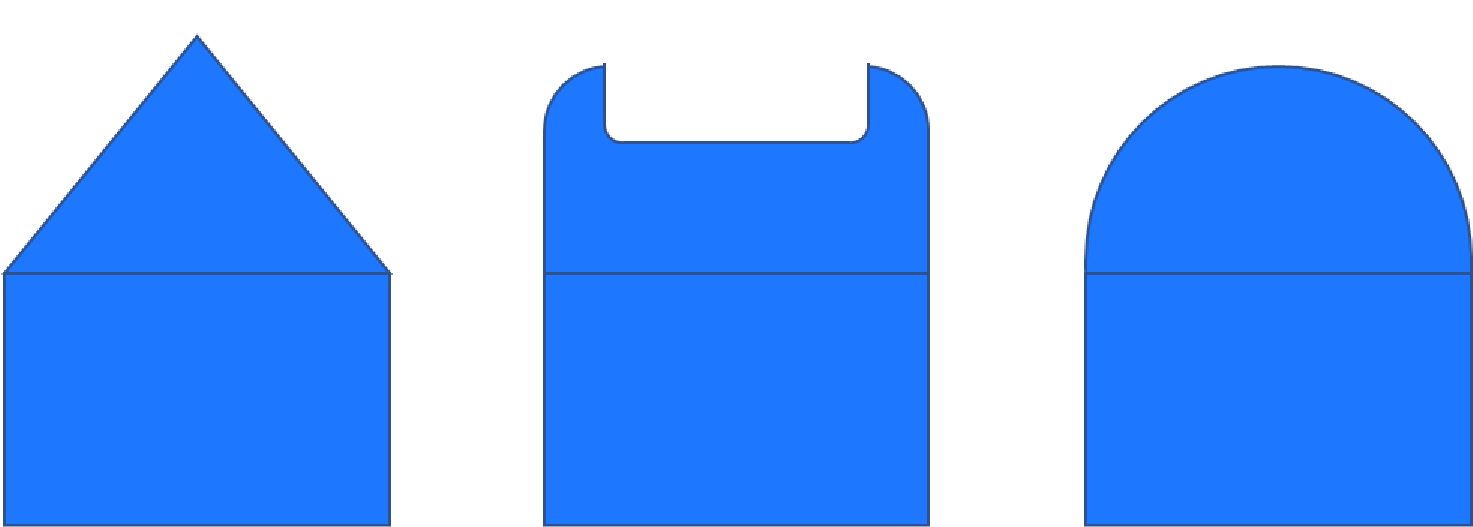
Figure 10: Sketches of Punches Used for Hole Expansion: Conical, Flat, and Hemispherical.
Metal motion and appearance changes depending on the type of punch used. Using a conical punch leads to the shape shown in Figure 11a, with a flat punch leading to the appearance shown in Figure 11b.S-3 The operations are sometimes described as hole expansion when accomplished with a conical punch, and hole extrusion with use of a flat punch.
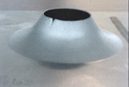
Figure 11a: Sample appearance after testing with conical punch.S-3
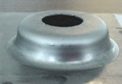
Figure 11b: Sample appearance after testing with flat punch.S-3
The ISO 16630 hole expansion test specifies the use of a conical punch with a 60 degree apex angle. Here, the free edge undergoes stretching and bending. Using a flat punch instead of a conical punch eliminates the bending component, and all deformation is from only edge stretching. These strain state differences lead to different sheared edge extension performance, with greater expansion prior to cracking achieved with holes expanded using a conical punch. This improved performance with conical rather than flat punches has been attributed to the presence of the bending component.N-10 Edge condition does not appear to influence hole expansion capability when a flat bottom punch is used.
Shown in Figures 12 to Figure 15 are the effects of burr orientation and punch type, which vary as a function of metal grade. Figure 16 compares the performance of reamed holes when expanded with either conical or flat punches. Where the tested grades perform similarly when expanded with a flat punch, the conical punch leads to exceptional performance of reamed holes of 3 of the 4 grades. The relatively poor performance of the DP780 grade may be due to the hardness differences between the ferrite and martensite components, noting that there is more martensite in DP780 than DP 600. In the study from which the data was taken, the complex phase steels had a yield/tensile ratio of approximately 87%, while for the dual phase grades the yield/tensile ratio was approximately 60%.P-13
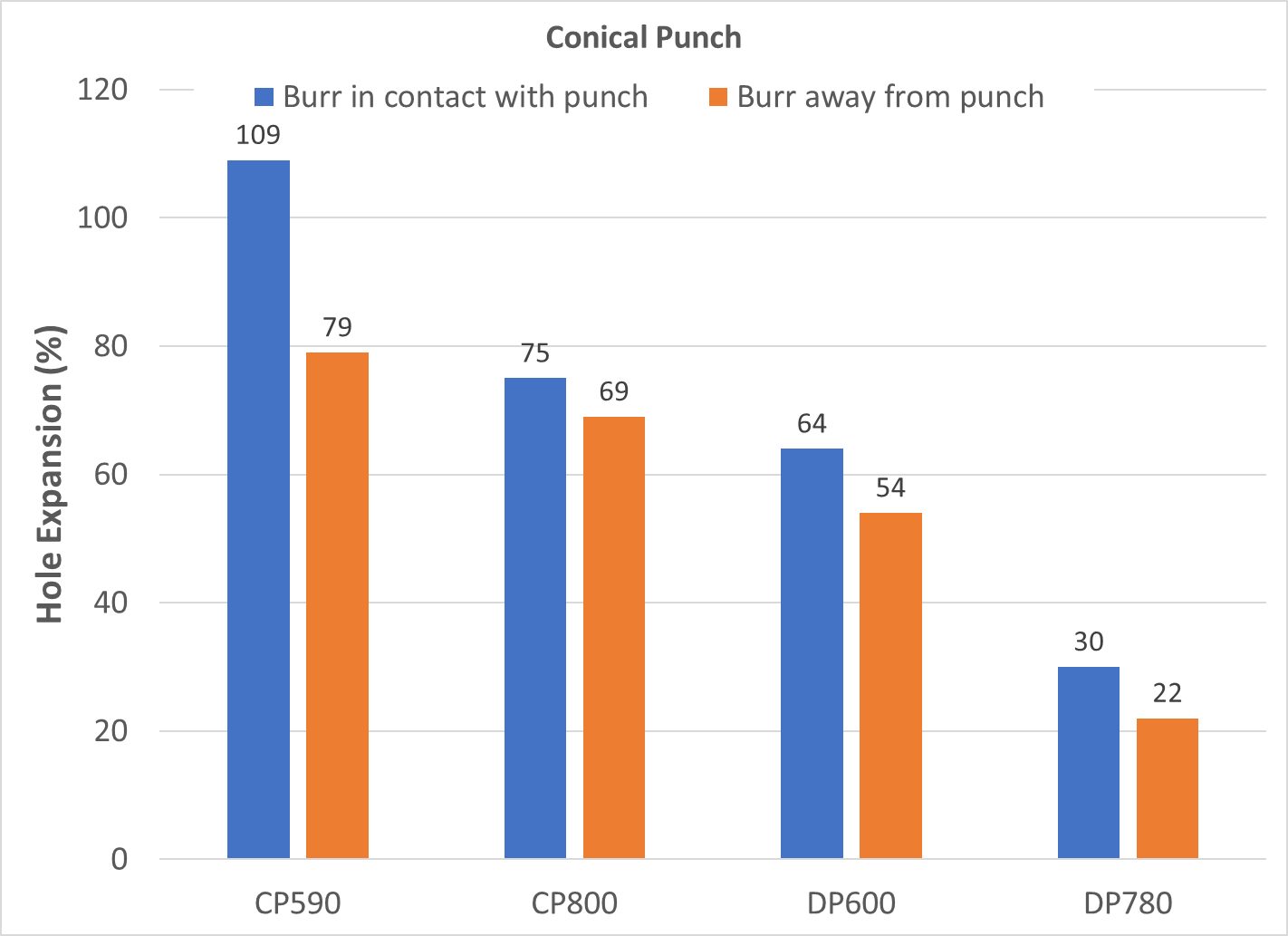
Figure 12: Effect of Burr Orientation on Hole Expansion from a Conical Punch. (Based on Data from Citation P-13.)
![Figure 13: Effect of Burr Orientation on Hole Expansion from a Flat Punch [Based on Data from Reference 11]](https://ahssinsights.org/wp-content/uploads/2020/07/Pasted-into-Hole-Expansion-Testing-Intro-31.jpg)
Figure 13: Effect of Burr Orientation on Hole Expansion from a Flat Punch. (Based on Data from Citation P-13.)
![Figure 14: Effect of Punch Type on Hole Expansion of Sheared Holes with Burr In Contact With The Punch [Based on Data from Reference 11]](https://ahssinsights.org/wp-content/uploads/2020/07/Pasted-into-Hole-Expansion-Testing-Intro-32.jpg)
Figure 14: Effect of Punch Type on Hole Expansion of Sheared Holes with Burr In Contact With The Punch. (Based on Data from Citation P-13.)
![Figure 15: Effect of Punch Type on Hole Expansion of Sheared Holes with Burr Facing Away From The Punch [Based on Data from Reference 11]](https://ahssinsights.org/wp-content/uploads/2020/07/Pasted-into-Hole-Expansion-Testing-Intro-33.jpg)
Figure 15: Effect of Punch Type on Hole Expansion of Sheared Holes with Burr Facing Away From The Punch. (Based on Data from Citation P-13.)
![Figure 16: Effect of Punch Type on Hole Expansion of Reamed Holes [Based on Data from Reference 11]](https://ahssinsights.org/wp-content/uploads/2020/07/Pasted-into-Hole-Expansion-Testing-Intro-34.jpg)
Figure 16: Effect of Punch Type on Hole Expansion of Reamed Holes. (Based on Data from Citation P-13.)
Figure 17 compares the simulation results from expanding a perfect edge (no burr, no strain) with a conical punch on the left and a spherical punch on the right.W-2 The color scale, based on a “damage” parameter, shows that a spherical punch results in a more uniform distribution of damage, especially at the edge. This suggests that the impact of burr orientation on hole expansion is less significant for this punch geometry.
Flanging with a conical punch causes high circumferential strain and high damage values at the outer edge. The inner edge of the sheet initially presses against the punch, and later stretches during flanging. Since cracks initiate at the fracture zone, using a conical punch with the burr facing the punch leads to a greater hole expansion capability than when having the burr in contact with a spherical punch.
Fracture initiates at the edge, and orienting the burr so that it is in contact with the punch leads to a greater hole expansion value.

Figure 17: Distribution of damage values in simulated hole expansion tests conducted with a conical punch (left image) and a hemispherical punch (right image).W-2
Improving Hole Expansion with New Punch Shapes
As explained above, the degree to which a sheared edge can be stretched before fracture is a function of many parameters, including the shape of the punch. Also contributing is the hardness uniformity of the microstructural phases, where grades with components having high hardness differences are associated with relatively lower hole expansion capability.
Researchers evaluated the effects of punch design and clearance on hole expansion capability of dual phase and ferrite-bainite steels, each with a tensile strength of approximately 780 MPa.L-47
In addition to a conventional flat punch face, other punch types studied were those with a beveled face, a humped shape, and a newly designed punch which combines the benefits of the prior two types. A chamfered or beveled punch is known to reduce punch forces and reverse snap-through loads, while at the same time improve edge quality and hole expansion by minimizing the hardness increases found in the shear affected zone.
Use of the humped punch (Figure 18) led to hole expansion improvements of up to 10%, compared with a conventional flat punch when testing either the DP or FB products. When comparing the edge characteristics, the humped punch results in an increased rollover zone. The authors attributed this to the hump geometry imposing axial tension on the steel during punching, thereby increasing stress triaxiality. Increases in stress triaxiality results in a reduction in effective stress even at the same average stress. This in turn lowers the plastic deformation at the sheared edge which minimizes edge fracture. For these reasons, the increases in stress triaxiality associated with the humped punch promotes higher levels of hole expansion.

Figure 18: Humped punch design used in Citation L-47.
A newly designed punch which combines a beveled and humped design (Figure 19) increases hole expansion by more than 30% in both dual phase and ferrite-bainite steels (Figure 20). As explained above, the newly designed punch is effective in promoting stress triaxiality and minimizing the plastic deformation near the sheared edge. Furthermore, the beveled design improves the shear affected zone (SAZ) characteristics, leading to improved sheared edge expandability as measured in a hole expansion test.

Figure 19: New punch design incorporating features of beveled and humped punches.L-47
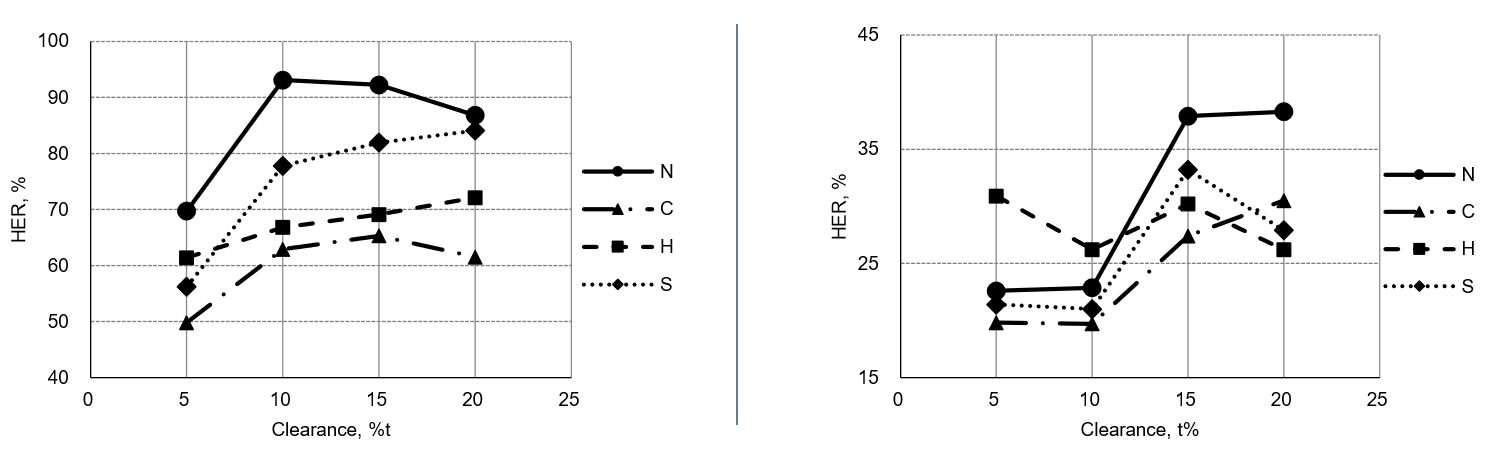
Figure 20: Effect of punch type and clearance on the hole expansion ratio of 780 MPa tensile strength steels. Left graph represents ferrite-bainite steel; right graph represents a dual phase steel. Legend: N=new punch design; C=conventional; H=humped; S=shear (beveled).L-47
Correlation of Hole Expansion Ratio with Tensile Properties
The complexities of hole expansion testing, as well as relatively few laboratories with the necessary test equipment and expertise, have led researchers to look for a correlation between the hole expansion ratio and conventionally measured properties obtained from a tensile test like the yield and tensile strength, uniform and total elongation, n-value, and r-value. Researchers even studied manipulations such as the yield-to-tensile ratio, tensile strength multiplied by uniform elongation, and n-value multiplied by r-value. Unfortunately, none of these properties or combinations have suitable correlation with the hole expansion ratio.
Recent work has shown a promising correlation between the hole expansion ratio and the true thinning strain at fracture. Our article on true fracture strain, describes this in greater detail.
Cutting, Cutting-Blanking-Shearing-Trimming
A cut edge has four distinct zones with different characteristics:
- Rollover – The plastically deformed zone bent as the cutting tools contact the edge of the sheet surface.
- Burnish – The zone where the cutting tools penetrate into the sheet metal, prior to any fracturing. The sheet metal stresses are such that the surface compresses into the cutting tool, which gives it a flat, smooth, and shiny appearance. The shear zone is another name for this region.
- Fracture – The zone where the cutting steels fracture the sheet metal, leading to separation from the remainder of the sheet. The surface of this region is rougher than the burnish zone, and is at an angle from the cutting direction.
- Burr – The metal elongated and pushed out on the trailing edge of the cut.
The cutting process also deforms the metal near the shear face, creating what is known as the shear affected zone (SAZ). The degree and influence of this deformation is a function of the cutting process (shearing, water jet, laser, and so on) as well as the steel microstructure and strength. Many studies conclude plastic deformation within the SAZ is a key contributor to sheared edge stretching failures.
The Shear Affected Zone is the area of work-hardened steel and microstructural damage behind the sheared edge. The deformation pattern in the SAZ includes a large shear-induced rotation of the grains that increases with proximity to the sheared edge. An etched cross-section such as that shown in Figure 1 highlights the grain rotation and gives a visual indication of the size of the zone.

Figure 1: Shear Face Components and Shear Affected Zone.K-1
Work hardening in the SAZ leads to a way to quantify the depth of the SAZ: by creating a hardness profile with readings starting at the edge and progressing deeper into the metal. The end of the SAZ occurs when the hardness readings level off to the bulk hardness.
Figure 2 compares the edge and hardness readings for CP800 and DP780, showing the depth of the SAZ for DP780 is about 41% of the initial sheet thickness of 1.56 mm, and the depth of the CP800 SAZ is 20% of the initial 2.90 mm sheet thickness.P-12

Figure 2: A) Sheared edge and SAZ of CP800; B) Sheared edge and SAZ of DP780; C) CP800 microstructure away from edge; D) DP780 microstructure away from sheared edge; E) hardness profile for the two sheared edges in the rolling direction.P-12
Plastic deformation from cutting and subsequent edge expansion forms micro-voids and creates other microstructural damage. The voids grow and combine with neighboring voids to create micro-cracks, which in turn combine with other micro-cracks resulting in the cracks that cause fractures in stampings.
Void nucleation in DP steels occurs through two mechanisms: decohesion of the ferrite-martensite interface or fracture of martensite islands.P-12, A-27, A-28 Figure 3 shows an example of both.

Figure 3: Aligned voids along loading direction. White solid square shows interface damage between martensite and ferrite. White dashed circles show voids formed by cracking of martensite.A-28
The study in Citation P-12 compared CP800 and DP780. The CP800 microstructure contains ferrite, bainite, and martensite. The DP780 microstructure has more martensite, with a larger strength differential between the phases, which combines to result in a lower nucleation strain and accelerated void nucleation compared with CP800. The DP grade has a larger SAZ, further promoting nucleation, growth, and coalescence of voids. This results in failure at a lower strain and leads to the lower edge stretchability of the DP780 compared to the CP800 alloy.P-12 The ductility of bainite restrains void initiation at high strains, which may play a role in improved sheared edge performance.S-24, S-25
Fracture initiation energy, a measure of fracture toughness correlates with hole expansion and stretch-flangeability as shown in Figure 4.Y-5

Figure 4: Correlation between fracture initiation energy and hole expansion ratio of various metals. Steels A – D are AHSS grades with Tensile strength ranging from 725 – 1000 MPa.Y-5
In addition to microstructural damage and fracture mechanics, simulation models improve in accuracy when the incorporating the effect of the temperature increase in the localized deformation zone. Simulation of the blanking of AISI 1050 shows a temperature at the cut edge of 440 °C. The edge temperature may be even higher in AHSS grades due to their higher strength.A-29

Figure 5: 1.27mm AISI 1050 steel blanked with 15% clearance. The simulation shows temperature, with the cut face getting as hot as 440 °C.A-29
When factoring in the considerations described here, simulation accuracy of hole expansion and sheared edge stretching improves significantly. Citations L-13 and L-14 provide additional background information about the Shear Affected Zone.