Blog, homepage-featured-top, main-blog
Equipment, Responsibilities, and Property Development Considerations When Deciding How A Part Gets Formed
Automakers contemplating whether a part is cold stamped or hot formed must consider numerous ramifications impacting multiple departments. Over a series of blogs, we’ll cover some of the considerations that must enter the discussion.
The discussions relative to cold stamping are applicable to any forming operation occurring at room temperature such as roll forming, hydroforming, or conventional stamping. Similarly, hot stamping refers to any set of operations using Press Hardening Steels (or Press Quenched Steels), including those that are roll formed or fluid-formed.
Equipment
There is a well-established infrastructure for cold stamping. New grades benefit from servo presses, especially for those grades where press force and press energy must be considered. Larger press beds may be necessary to accommodate larger parts. As long as these factors are considered, the existing infrastructure is likely sufficient.
Progressive-die presses have tonnage ratings commonly in the range of 630 to 1250 tons at relatively high stroke rates. Transfer presses, typically ranging from 800 to 2500 tons, operate at relatively lower stroke rates. Power requirements can vary between 75 kW (630 tons) to 350 kW (2500 tons). Recent transfer press installations of approximately 3000 tons capacity allow for processing of an expanded range of higher strength steels.
Hot stamping requires a high-tonnage servo-driven press (approximately 1000 ton force capacity) with a 3 meter by 2 meter bolster, fed by either a roller-hearth furnace more than 30 m long or a multi-chamber furnace. Press hardened steels need to be heated to 900 °C for full austenitization in order to achieve a uniform consistent phase, and this contributes to energy requirements often exceeding 2 MW.
Integrating multiple functions into fewer parts leads to part consolidation. Accommodating large laser-welded parts such as combined front and rear door rings expands the need for even wider furnaces, higher-tonnage presses, and larger bolster dimensions.
Blanking of coils used in the PHS process occurs before the hardening step, so forces are low. Post-hardening trimming usually requires laser cutting, or possibly mechanical cutting if some processing was done to soften the areas of interest.
That contrasts with the blanking and trimming of high strength cold-forming grades. Except for the highest strength cold forming grades, both blanking and trimming tonnage requirements are sufficiently low that conventional mechanical cutting is used on the vast majority of parts. Cut edge quality and uniformity greatly impact the edge stretchability that may lead to unexpected fracture.
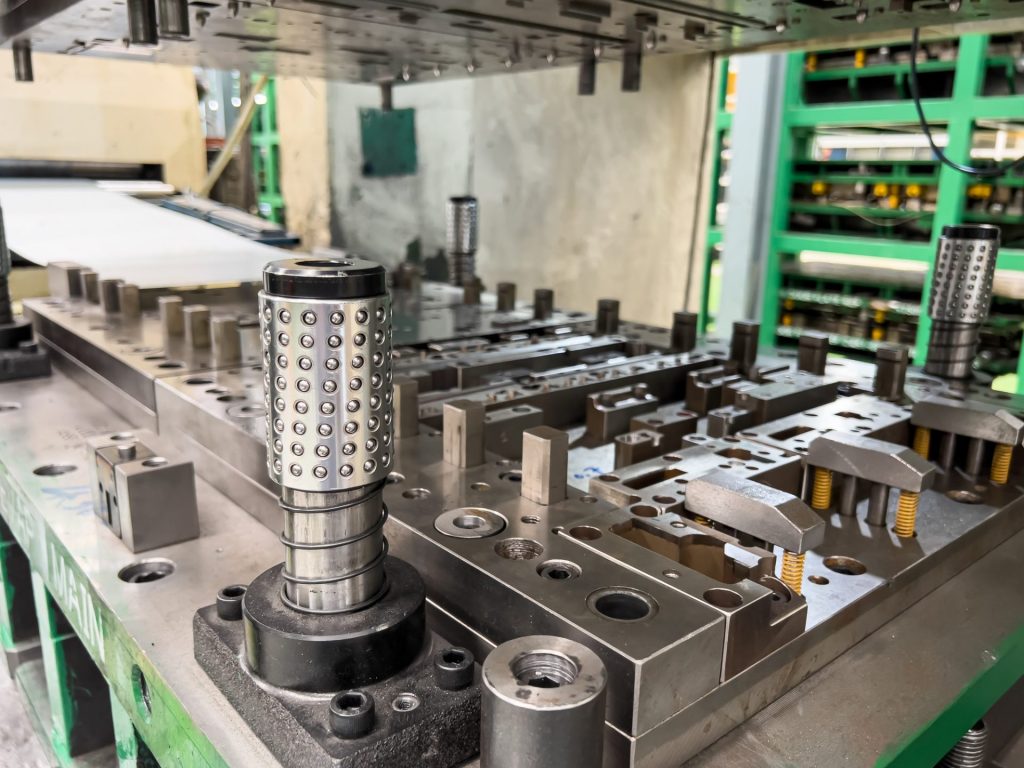
Responsibilities
Most cold stamped parts going into a given body-in-white are formed by a tier supplier. In contrast, some automakers create the vast majority of their hot stamped parts in-house, while others rely on their tier suppliers to provide hot stamped components. The number of qualified suppliers capable of producing hot stamped parts is markedly smaller than the number of cold stamping part suppliers.
Hot stamping is more complex than just adding heat to a cold stamping process. Suppliers of cold stamped parts are responsible for forming a dimensionally accurate part, assuming the steel supplier provides sheet metal with the required tensile properties achieved with a targeted microstructure.
Suppliers of hot stamped parts are also responsible for producing a dimensionally accurate part, but have additional responsibility for developing the microstructure and tensile properties of that part from a general steel chemistry typically described as 22MnB5.
Property Development
Independent of which company creates the hot formed part, appropriate quality assurance practices must be in place. With cold stamped parts, steel is produced to meet the minimum requirements for that grade, so routine property testing of the formed part is usually not performed. This is in contrast to hot stamped parts, where the local quench rate has a direct effect on tensile properties after forming. If any portion of the part is not quenched faster than the critical cooling rate, the targeted mechanical properties will not be met and part performance can be compromised. Many companies have a standard practice of testing multiple areas on samples pulled every run. It’s critical that these tested areas are representative of the entire part. For example, on the top of a hat-section profile where there is good contact between the punch and cavity, heat extraction is likely uniform and consistent. However, on the vertical sidewalls, getting sufficient contact between the sheet metal and the tooling is more challenging. As a result, the reduced heat extraction may limit the strengthening effect due to an insufficient quench rate.
For more information, see our Press Hardened Steel Primer to learn more about PHS grades and processing!
Thanks are given to Eren Billur, Ph.D., Billur MetalForm for his contributions to the Equipment section, as well as many of the webpages relating to Press Hardening Steels at www.AHSSinsights.org.
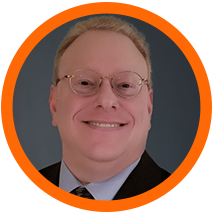
Danny Schaeffler is the Metallurgy and Forming Technical Editor of the AHSS Applications Guidelines available from WorldAutoSteel. He is founder and President of Engineering Quality Solutions (EQS). Danny wrote the monthly “Science of Forming” and “Metal Matters” column for Metalforming Magazine, and provides seminars on sheet metal formability for Auto/Steel Partnership and the Precision Metalforming Association. He has written for Stamping Journal and The Fabricator, and has lectured at FabTech. Danny is passionate about training new and experienced employees at manufacturing companies about how sheet metal properties impact their forming success.
Coatings
topofpage
Many formed parts require corrosion protection, achieved through the application of some type of zinc-based or aluminum-based coating. The primary methods of applying zinc are through a hot dipped galvanizing line, or through an electro-galvanizing process. Aluminum-based coatings are applied in a hot dipped aluminizing line.
Both aluminized and galvanized coatings provide a barrier layer preventing corrosion of the underlying sheet steel. Zinc-based coatings also provide galvanic protection, where the zinc acts as a sacrificial anode if scratches or impact damage the coating, and therefore corrodes first before the underlying steel.
Aluminum melts at a higher temperature than zinc, which is why aluminized steels are more frequently used in applications requiring corrosion protection at elevated temperatures such as those found in the engine compartment and in the exhaust system. There are two types of aluminized coatings, known as Type 1 (aluminum with 9% silicon) and Type 2 (pure aluminum). The aluminum-silicon AlSi coating associated with press hardening steels are these Type 1 aluminized coatings. There are limited automotive applications for Type 2 pure aluminum coatings.
Zinc-magnesium coatings are relatively new options, offering enhanced cut edge corrosion protection, with lower friction and lower risk of galling and powdering than other common zinc coatings.
Most hot dipped galvanized lines produce surfaces which result in similar coefficients of friction for a given steel type. Different electrogalvanized coating lines may result in different surface morphologies, which can result in significantly different formability characteristics.
Passing a pure zinc-coated steel through a furnace allows the steel and zinc to inter-diffuse and results in an alloyed coating known as galvanneal. Hot dipped galvannealed coatings have improved joining due to the iron in the coating. Unlike the uniform coating composition associated with hot dip galvanized and electrogalvanized coatings, GA coatings are composed of different phases with varying composition. This may lead to different forming, joining, and painting characteristics when comparing products produced on different lines.
Differences in performance due to coating line characteristics is another reason why it is good practice to Identify the intended steel production source early in the die construction and die try-out process. Tryout material should come from the same source as will supply production.
Hot Dipped Galvanized and Galvannealed Coatings
The majority of sheet steel parts on a vehicle require corrosion protection, independent of whether they are made from mild or high strength steel and whether they are intended for exposed or unexposed applications. Hot dipped galvanizing – applying a zinc coating over the steel – is the most common way to achieve corrosion protection. It is an economical solution, since cold rolled steel can be annealed and coated in the same continuous operation.
A typical in-line continuous hot dip galvanizing line such as that shown in Figure 1 uses a full-hard cold rolled steel coil as the feedstock. Welding individual coils together produces a continuous strip. After cleaning, the strip is processed in a continuous annealing furnace where the microstructure is recrystallized, improving forming characteristics. Adjustments to the annealing temperature produces the desired microstructure associated with the ordered grade. Rather than cooling to room temperature, the in-process coil is cooled to just above 460 °C (860 °F), the temperature of the molten zinc bath it enters. The chemistry in the zinc pot is a function of whether a hot dipped galvanized or galvannealed coating is ordered. Hot rolled steels may be coated with the hot dip galvanizing process, but different processing conditions are used to achieve the targeted properties.

Figure 1: Schematic of a typical hot dipped galvanizing line with galvanneal capability.
There are several types of hot dipped coatings for automotive applications, with unique characteristics that affect their corrosion protection, lubricity for forming, weldability and paintability. One of the primary hot dipped galvanized coatings is a pure zinc coating (abbreviated as GI), sometime referred to as free zinc. The molten zinc bath has small amounts of aluminum which helps to form a thin Fe2Al5 layer at the zinc-steel interface. This thin barrier layer prevents zinc from diffusing into the base steel, which leaves the coating as essentially pure zinc.
Coils pass through the molten zinc at speeds up to 3 meters per second. Zinc coating weight is controlled by gas knives (typically air or nitrogen) blowing off excess liquid zinc as the coil emerges from the bath. Zinc remaining on the surface solidifies into crystals called spangle. Molten zinc chemistry and cooling practices used at the galvanizing line control spangle size. In one extreme, a large spangle zinc coating is characteristic of garbage cans and grain silos. In the other extreme, the zinc crystal structure is sufficiently fine that it is not visible to the unaided eye. Since spangle can show through on a painted surface, a minimum-spangle or no-spangle option is appropriate for surface-critical applications.
The other primary hot dipped coating used for corrosion protection is hot dipped galvanneal (abbreviated as GA). Applying this coating to a steel coil involves the same steps as creating a free zinc hot dipped coated steel, but after exiting the zinc pot, the steel strip passes through a galvannealing furnace where the zinc coating is reheated while still molten.
The molten zinc bath used to produce a GA coating has a lower aluminum content than what is used to produce a GI coating. Without aluminum to create the barrier layer, the zinc coating and the base steel inter-diffuse freely, creating an iron-zinc alloy with typical average iron content in the 8% to 12% range. The iron content improves weldability, which is a key attribute of the galvanneal coatings.
The iron content is unevenly distributed throughout the coating, ranging from 5% at the surface (where the sheet metal coating contacts the tool surface during forming) to as much as 25% iron content at the steel/coating interface. The amount of iron at the surface and distribution within the coating is a function of galvannealing parameters and practices – primarily the bath composition and time spent at the galvannealing temperature. Coating iron content impacts coating hardness, which affects the interaction with the sheet forming lubricant and tools, and results in changes in friction. The hard GA coatings have a greater powdering tendency during contact with tooling surfaces, especially during movement through draw beads. Powdering is minimized by using thinner coatings – where 50 g/m2 to 60 g/m2 (50G to 60G) is a typical EG and GI coating weight, GA coatings are more commonly between 30 g/m2 to 45 g/m2 (30A to 45A)
Options to improve formability on parts made from GA coated steels include use of press-applied lubricants or products that can be applied at the steel mill after galvanizing, like roll-coated phosphate, which have the additional benefit of added lubricity. The surface morphology of a galvannealed surface (Figure 2) promotes good phosphate adherence, which in turn is favorable for paintability.

Figure 2: High magnification photograph of a galvannealed steel surface. The surface structure results in excellent paint adhesion.
Galvannealed coatings provides excellent corrosion protection to the underlying steel, as do GI and EG coatings. GI and EG coatings are essentially pure zinc. Zinc acts as a sacrificial anode if scratches or impact damages either coating, and therefore will corrode first before the underlying steel. The corrosion product of GI and EG is white, and is a combination of zinc carbonate and zinc hydroxide. A similar mechanism protects GA coated steels, but the presence of iron in the coating may result in a reddish tinge to the corrosion product. This should not be interpreted as an indication of corrosion of the steel substrate.
Another option is to change the bath composition such that it contains proper amounts of aluminum and magnesium. The results in a zinc-magnesium (ZM) coating, which has excellent cut edge corrosion protection.
Producing galvanized and galvannealed Advanced High Strength Steels is challenging due to the interactions of the necessary thermal cycles at each step. As an example, the targeted microstructure of Dual Phase steels can be achieved by varying the temperature and time the steel strip passes through the zinc bath, and can be adjusted to achieve the targeted strength level. However, not all advanced high strength steels can attain their microstructure with the thermal profile of a conventional hot dipped galvanizing line with limited rapid quenching capabilities. In addition, many AHSS grades have chemistries that lead to increased surface oxides, preventing good zinc adhesion to the surface. These grades must be produced on a stand-alone Continuous Annealing Line, or CAL, without an in-line zinc pot. Continuous Annealing Lines feature a furnace with variable and rapid quenching operations that enable the thermal processing required to achieve very high strength levels. If corrosion protection is required, these steel grades are coated on an electrogalvanizing line (EG) in a separate operation, after being processed on a CAL line.
Hot dipped galvanizing lines at different steel companies have similar processes that result in similar surfaces with respect to coefficient of friction. Surface finish and texture (and resultant frictional characteristics) are primarily due to work roll textures, based on the customer specification. Converting from one coating line to another using the same specification is usually not of major significance with respect to coefficient of friction. A more significant change in friction is observed with changes between GI and GA and EG.
Electrogalvanized Coatings
Electrogalvanizing is a zinc deposition process, where the zinc is electrolytically bonded to steel in order to protect against corrosion. The process involves electroplating: running an electrical current through the steel strip as it passes through a saline/zinc solution.
Electrogalvanizing occurs at room temperature, so the microstructure, mechanical, and physical properties of AHSS products achieved on a continuous anneal line (CAL) are essentially unchanged after the electrogalvanizing (EG) process. EG lines have multiple plating cells, with each cell capable of being on or off. As a result, chief advantages of electrogalvanizing compared to hot dipped galvanizing include: (1) lower processing temperatures, (2) precise coating weight control, and (3) brighter, more uniform coatings which are easier to convert to Class A exposed quality painted surfaces.
The majority of electrogalvanizing lines can apply only pure (free) zinc coatings, known as EG for electrogalvanized steel. Selected lines can apply different types of coatings, like EGA (electro-galvanneal) or Zn-Ni (zinc-nickel).
There are no concerns about different alloy phases in the coating as with galvanneal coatings. The lack of aluminum in the coating results in improved weldability. The biggest concern with electrogalvanizing lines is the coefficient of friction. Electrogalvanized (EG) coatings have a relatively high coefficient of friction – higher than hot dipped galvanized coatings, but lower than galvanneal coatings. To improve formability of electrogalvanized sheets, some automakers choose to use a steel mill-applied pre-lube rather than a simple mill-applied rust preventive oil.
A representative EG line is shown in Figure 3. Different EG lines may use different technologies to apply the zinc crystals. Because the zinc crystals are deposited in a different fashion, these different processes may potentially result in different surface morphology and, in turn, a different coefficient of friction. Dry conditions may result in a higher coefficient of friction, but the “stacked plate-like surface morphology” (Figure 4) allows these coatings to trap and hold lubrication better than the smoother surfaces of hot dipped galvanizing coatings. Auto manufacturers should therefore consult the steel supplier for specific lubricant recommendations based on the forming needs.

Figure 3: Schematic of an electrogalvanizing line.

Figure 4: High magnification photograph of electrogalvanized steel surface showing stacked plate-like structure.
Zinc-Magnesium (ZM) Coatings
Galvanized coatings offer excellent corrosion protection to the underlying steel. Each type of galvanized coating has characteristics that make it suitable for specific applications and environments. The method by which the galvanization occurs (electrolytically applied vs. hot dipping) changes these characteristics, as does the coating chemistry (pure zinc vs. zinc alloy)
Adding small amounts of magnesium and aluminum affects the coating properties, which influences friction, surface appearance, and corrosion among other parameters.
Industries like agriculture and construction have used a coating known as ZAM for several decades. ZAM (Zinc-Aluminum-Magnesium) is primarily zinc, with approximately 6% Al and 3% Mg. However, the high aluminum content does not lend itself to a continuous galvanizing operation due to increased dross formation. The coating aluminum also degrades weldability.
A different coating, known as ZM, was commercialized around 2010. This zinc-rich coating typically has 1% to 3% of both magnesium and aluminum, with some companies using a slightly higher amount of aluminum. ZM coatings are typically applied using a hot dip approach like GI and GA, but with an appropriate bath chemistry. Exposed quality surfaces are achievable.
Even though ZM is a relatively hard coating, it is associated with lower friction and lower risk of galling and powdering than other common zinc coatings. This allows for parts to be successfully formed using higher blank holder forces, resulting in a wider BHF range between wrinkles and splits.Z-6
ZM coatings have similar joining characteristics and similar performance after phosphating / painting as hot dip galvanized coatings.
Enhanced cut edge corrosion protection relative to EG, GI, and GA coatings occurs due to the formation of a stable passivation layer on the bare steel edge that would be otherwise exposed to the environment. ZM coating corrosion dynamics are such that similar performance to EG, GI, and GA can be achieved at lower ZM coating weights.
Powdering resistance of ZM coatings are similar to that for GI and EG, and better than what is seen on GA coatings. ZM coatings have superior cyclic, perforating, and stone chip corrosion resistance.
Potential areas of application applications include:
- Hem flanges of doors, deck lids, and hoods
- Cut edges in inner hood, door, and deck lid panels
- Stone chip sensitive parts like hoods, fenders, doors, and body sides.
- Difficult to form parts that can benefit from the lower friction
main-blog
Many steel parts on a vehicle require corrosion protection, regardless of whether they are exposed or unexposed applications. The most common way to accomplish corrosion protection is to coat Advanced High-Strength Steels (AHSS) with zinc by means of a couple of different processes. This AHSS Insights Blog goes over the most common.
Electrogalvanizing
Electrogalvanizing is a zinc deposition process, where the zinc is electrolytically bonded to steel in order to protect against corrosion. The process involves electroplating: running an electrical current through the steel strip as it passes through a saline/zinc solution. Electrogalvanizing is done at room temperature, so the microstructure, mechanical, and physical properties of AHSS products achieved on a continuous anneal line (CAL) are essentially unchanged after the electrogalvanizing (EG) process. EG lines have multiple plating cells, with each cell capable of being on or off. As a result, chief advantages of electrogalvanizing compared to hot dipped galvanizing include: (1) lower processing temperatures, (2) precise coating weight control, and (3) brighter, more uniform coatings which are easier to convert to Class A exposed quality painted surfaces.
The majority of electrogalvanizing lines can apply only pure (free) zinc coatings, known as EG for electrogalvanized steel. Selected lines can apply different types of coatings, like EGA (electro-galvanneal) or Zn-Ni (zinc-nickel).
There are no concerns about different alloy phases in the coating as with galvanneal coatings. The lack of aluminum in the coating results in improved weldability. The biggest concern with electrogalvanizing lines is the coefficient of friction. Electrogalvanized (EG) coatings have a relatively high coefficient of friction—higher than hot dipped galvanized coatings, but lower than galvanneal coatings. To improve formability of electrogalvanized sheets, some automakers choose to use a steel mill-applied pre-lube rather than a simple mill-applied rust preventive oil.
A representative EG line is shown in Figure 1. Different EG lines may use different technologies to apply the zinc crystals. Because the zinc crystals are deposited in a different fashion, these different processes may potentially result in different surface morphology and, in turn, a different coefficient of friction.

Figure 1: Schematic of an electrogalvanizing line.
A higher coefficient of friction may be found under dry conditions, but the “stacked plate-like surface morphology” (Figure 2) allows these coatings to trap and hold lubrication better than the smoother surfaces of hot dipped galvanizing coatings. Auto manufacturers should therefore consult the steel supplier for specific lubricant recommendations based on the forming needs.
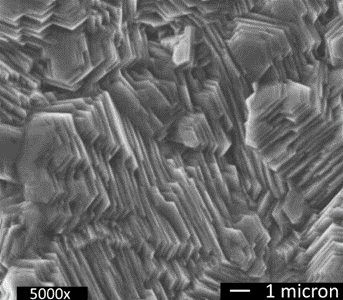
Figure 2: High magnification photograph of electrogalvanized steel surface showing stacked plate-like structure.
Hot Dip Galvanize and Hot Dip Galvanneal
Hot dipped galvanizing – applying a zinc coating over the steel – is the most common way to achieve corrosion protection. It is an economical solution, since cold rolled steel can be annealed and coated in the same continuous operation.
A typical in-line continuous hot dip galvanizing line such as that shown in Figure 3 uses a full-hard cold rolled steel coil as the feedstock. Individual coils are welded together to produce a continuous strip. After cleaning, the strip is processed in a continuous annealing furnace where the microstructure is recrystallized, improving forming characteristics. The annealing temperature is adjusted to produce the desired microstructure associated with the ordered grade. Rather than cooling to room temperature, the in-process coil is cooled to just above 460°C (860°F), the temperature of the molten zinc bath it enters. The chemistry in the zinc pot is a function of whether a hot dipped galvanized or galvannealed coating is ordered. Hot rolled steels also are coated with the hot dip galvanizing process, but different processing conditions are used to achieve the targeted properties.
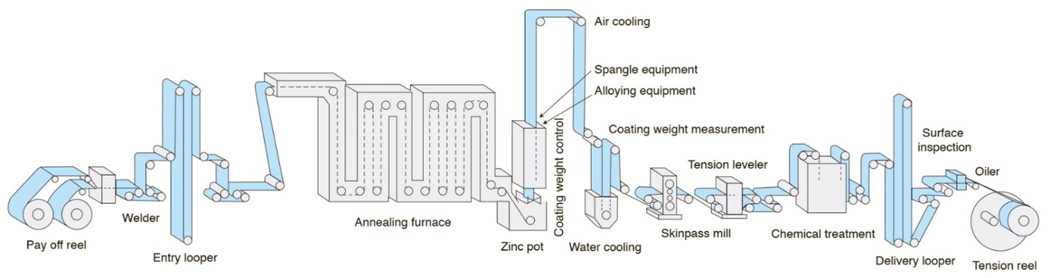
Figure 3: Schematic of a typical hot dipped galvanizing line with galvanneal capability.
There are several types of hot dipped coatings for automotive applications, with unique characteristics that affect their corrosion protection, lubricity for forming, weldability and paintability. One of the primary hot dipped galvanized coatings is a pure zinc coating (abbreviated as GI), sometime referred to as free zinc. The molten zinc bath has small amounts of aluminum which helps to form a thin Fe2Al5 layer at the zinc-steel interface. This thin barrier layer prevents zinc from diffusing into the base steel, which leaves the coating as essentially pure zinc.
Coil pass through the molten zinc at speeds up to 3 meters per second. Zinc coating weight is controlled by gas knives (typically air or nitrogen) blowing off excess liquid zinc as the coil emerges from the bath. Zinc remaining on the surface solidifies into crystals called spangle. Molten zinc chemistry and cooling practices used at the galvanizing line control spangle size. Since spangle can show through on a painted surface, a minimum-spangle or no-spangle option is appropriate for surface-critical applications.
The other primary hot dipped coating used for corrosion protection is hot dipped galvanneal (abbreviated as GA). Applying this coating to a steel coil involves the same steps as creating a free zinc hot dipped coated steel, but after exiting the zinc pot, the steel strip passes through a galvannealing furnace where the zinc coating is reheated while still molten.
The molten zinc bath used to produce a GA coating has a lower aluminum content than what is used to produce a GI coating. Without aluminum to create the barrier layer, the zinc coating and the base steel inter-diffuse freely, creating an iron-zinc alloy with typical average iron content in the 8-12% range. The iron content improves weldability, which is a key attribute of the galvanneal coatings.
The iron content will be unevenly distributed throughout the coating, ranging from 5% at the surface (where the sheet metal coating contacts the tool surface during forming) to as much as 25% iron content at the steel/coating interface. The amount of iron at the surface and distribution within the coating is a function of galvannealing parameters and practices – primarily the bath composition and time spent at the galvannealing temperature. Coating iron content impacts coating hardness, which affects the interaction with the sheet forming lubricant and tools, and results in changes in friction. The hard GA coatings have a greater powdering tendency during contact with tooling surfaces, especially during movement through draw beads. Powdering is minimized by using thinner coatings – where 50 g/m2 to 60 g/m2 (50G to 60G) is a typical EG and GI coating weight, GA coatings are more commonly between 30 g/m2 to 45 g/m2 (30A to 45A).
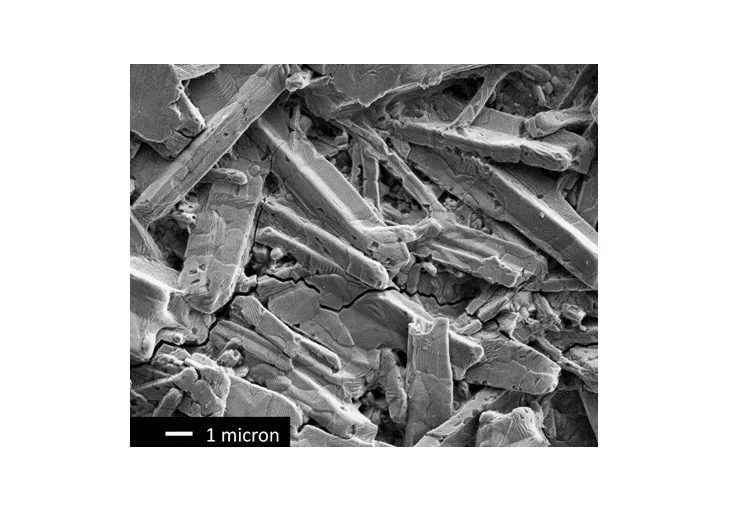
Figure 4: High magnification photograph of a galvannealed steel surface. The surface structure results in excellent paint adhesion.
Options to improve formability on parts made from GA coated steels include use of press-applied lubricants or products that can be applied at the steel mill after galvanizing, like roll-coated phosphate, which have the additional benefit of added lubricity. The surface morphology of a galvannealed surface (Figure 4) promotes good phosphate adherence, which in turn is favorable for paintability.
Galvannealed coatings provide excellent corrosion protection to the underlying steel, as do GI and EG coatings. GI and EG coatings are essentially pure zinc. Zinc acts as a sacrificial anode if either coating is damaged from scratches or impact, and therefore will corrode first before the underlying steel. The corrosion product of GI and EG is white and is a combination of zinc carbonate and zinc hydroxide. A similar mechanism protects GA coated steels, but the presence of iron in the coating may result in a reddish tinge to the corrosion product. This should not be interpreted as an indication of corrosion of the steel substrate.
Producing galvanized and galvannealed AHSS is challenging due to the interactions of the necessary thermal cycles at each step. As an example, the targeted microstructure of Dual Phase steels can be achieved by varying the temperature and time the steel strip passes through the zinc bath and can be adjusted to achieve the targeted strength level. However, not all AHSS can attain their microstructure with the thermal profile of a conventional hot dipped galvanizing line with limited rapid quenching capabilities. In addition, many AHSS grades have chemistries that lead to increased surface oxides, preventing good zinc adhesion to the surface. These grades must be produced on a stand-alone Continuous Annealing Line, or CAL, without an in-line zinc pot. Continuous Annealing Lines feature a furnace with variable and rapid quenching operations that enable the thermal processing required to achieve very high-strength levels. If corrosion protection is required, these steel grades are coated on an electrogalvanizing line (EG) in a separate operation, after being processed on a CAL line.
Hot dipped galvanizing lines at different steel companies have similar processes that result in similar surfaces with respect to coefficient of friction. Surface finish and texture (and resultant frictional characteristics) are primarily due to work roll textures, based on the customer specification. Converting from one coating line to another using the same specification is usually not of major significance with respect to coefficient of friction. A more significant change in friction is observed with changes between GI and GA and EG.
Soon, we’ll release the results of a three-year study on Liquid Metal Embrittlement in resistance spot welding. It will shed light on why LME occurs, how its occurrence can be controlled, and practical preventative measures to avoid LME on the manufacturing line. Stay tuned.