Many steel parts on a vehicle require corrosion protection, regardless of whether they are exposed or unexposed applications. The most common way to accomplish corrosion protection is to coat Advanced High-Strength Steels (AHSS) with zinc by means of a couple of different processes. This AHSS Insights Blog goes over the most common.
Electrogalvanizing
Electrogalvanizing is a zinc deposition process, where the zinc is electrolytically bonded to steel in order to protect against corrosion. The process involves electroplating: running an electrical current through the steel strip as it passes through a saline/zinc solution. Electrogalvanizing is done at room temperature, so the microstructure, mechanical, and physical properties of AHSS products achieved on a continuous anneal line (CAL) are essentially unchanged after the electrogalvanizing (EG) process. EG lines have multiple plating cells, with each cell capable of being on or off. As a result, chief advantages of electrogalvanizing compared to hot dipped galvanizing include: (1) lower processing temperatures, (2) precise coating weight control, and (3) brighter, more uniform coatings which are easier to convert to Class A exposed quality painted surfaces.
The majority of electrogalvanizing lines can apply only pure (free) zinc coatings, known as EG for electrogalvanized steel. Selected lines can apply different types of coatings, like EGA (electro-galvanneal) or Zn-Ni (zinc-nickel).
There are no concerns about different alloy phases in the coating as with galvanneal coatings. The lack of aluminum in the coating results in improved weldability. The biggest concern with electrogalvanizing lines is the coefficient of friction. Electrogalvanized (EG) coatings have a relatively high coefficient of friction—higher than hot dipped galvanized coatings, but lower than galvanneal coatings. To improve formability of electrogalvanized sheets, some automakers choose to use a steel mill-applied pre-lube rather than a simple mill-applied rust preventive oil.
A representative EG line is shown in Figure 1. Different EG lines may use different technologies to apply the zinc crystals. Because the zinc crystals are deposited in a different fashion, these different processes may potentially result in different surface morphology and, in turn, a different coefficient of friction.
A higher coefficient of friction may be found under dry conditions, but the “stacked plate-like surface morphology” (Figure 2) allows these coatings to trap and hold lubrication better than the smoother surfaces of hot dipped galvanizing coatings. Auto manufacturers should therefore consult the steel supplier for specific lubricant recommendations based on the forming needs.
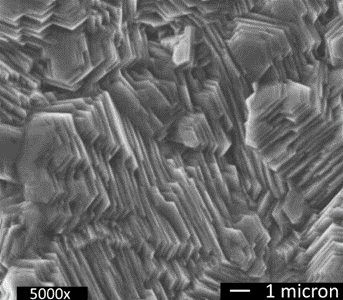
Figure 2: High magnification photograph of electrogalvanized steel surface showing stacked plate-like structure.
Hot Dip Galvanize and Hot Dip Galvanneal
Hot dipped galvanizing – applying a zinc coating over the steel – is the most common way to achieve corrosion protection. It is an economical solution, since cold rolled steel can be annealed and coated in the same continuous operation.
A typical in-line continuous hot dip galvanizing line such as that shown in Figure 3 uses a full-hard cold rolled steel coil as the feedstock. Individual coils are welded together to produce a continuous strip. After cleaning, the strip is processed in a continuous annealing furnace where the microstructure is recrystallized, improving forming characteristics. The annealing temperature is adjusted to produce the desired microstructure associated with the ordered grade. Rather than cooling to room temperature, the in-process coil is cooled to just above 460°C (860°F), the temperature of the molten zinc bath it enters. The chemistry in the zinc pot is a function of whether a hot dipped galvanized or galvannealed coating is ordered. Hot rolled steels also are coated with the hot dip galvanizing process, but different processing conditions are used to achieve the targeted properties.
There are several types of hot dipped coatings for automotive applications, with unique characteristics that affect their corrosion protection, lubricity for forming, weldability and paintability. One of the primary hot dipped galvanized coatings is a pure zinc coating (abbreviated as GI), sometime referred to as free zinc. The molten zinc bath has small amounts of aluminum which helps to form a thin Fe2Al5 layer at the zinc-steel interface. This thin barrier layer prevents zinc from diffusing into the base steel, which leaves the coating as essentially pure zinc.
Coil pass through the molten zinc at speeds up to 3 meters per second. Zinc coating weight is controlled by gas knives (typically air or nitrogen) blowing off excess liquid zinc as the coil emerges from the bath. Zinc remaining on the surface solidifies into crystals called spangle. Molten zinc chemistry and cooling practices used at the galvanizing line control spangle size. Since spangle can show through on a painted surface, a minimum-spangle or no-spangle option is appropriate for surface-critical applications.
The other primary hot dipped coating used for corrosion protection is hot dipped galvanneal (abbreviated as GA). Applying this coating to a steel coil involves the same steps as creating a free zinc hot dipped coated steel, but after exiting the zinc pot, the steel strip passes through a galvannealing furnace where the zinc coating is reheated while still molten.
The molten zinc bath used to produce a GA coating has a lower aluminum content than what is used to produce a GI coating. Without aluminum to create the barrier layer, the zinc coating and the base steel inter-diffuse freely, creating an iron-zinc alloy with typical average iron content in the 8-12% range. The iron content improves weldability, which is a key attribute of the galvanneal coatings.
The iron content will be unevenly distributed throughout the coating, ranging from 5% at the surface (where the sheet metal coating contacts the tool surface during forming) to as much as 25% iron content at the steel/coating interface. The amount of iron at the surface and distribution within the coating is a function of galvannealing parameters and practices – primarily the bath composition and time spent at the galvannealing temperature. Coating iron content impacts coating hardness, which affects the interaction with the sheet forming lubricant and tools, and results in changes in friction. The hard GA coatings have a greater powdering tendency during contact with tooling surfaces, especially during movement through draw beads. Powdering is minimized by using thinner coatings – where 50 g/m2 to 60 g/m2 (50G to 60G) is a typical EG and GI coating weight, GA coatings are more commonly between 30 g/m2 to 45 g/m2 (30A to 45A).
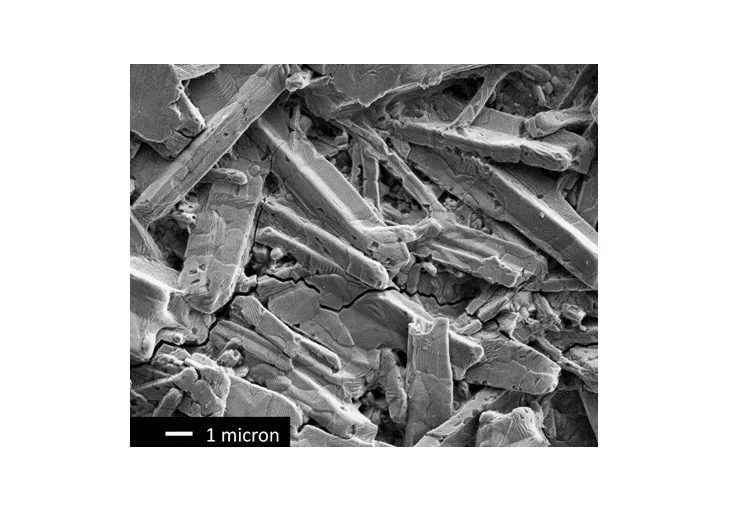
Figure 4: High magnification photograph of a galvannealed steel surface. The surface structure results in excellent paint adhesion.
Options to improve formability on parts made from GA coated steels include use of press-applied lubricants or products that can be applied at the steel mill after galvanizing, like roll-coated phosphate, which have the additional benefit of added lubricity. The surface morphology of a galvannealed surface (Figure 4) promotes good phosphate adherence, which in turn is favorable for paintability.
Galvannealed coatings provide excellent corrosion protection to the underlying steel, as do GI and EG coatings. GI and EG coatings are essentially pure zinc. Zinc acts as a sacrificial anode if either coating is damaged from scratches or impact, and therefore will corrode first before the underlying steel. The corrosion product of GI and EG is white and is a combination of zinc carbonate and zinc hydroxide. A similar mechanism protects GA coated steels, but the presence of iron in the coating may result in a reddish tinge to the corrosion product. This should not be interpreted as an indication of corrosion of the steel substrate.
Producing galvanized and galvannealed AHSS is challenging due to the interactions of the necessary thermal cycles at each step. As an example, the targeted microstructure of Dual Phase steels can be achieved by varying the temperature and time the steel strip passes through the zinc bath and can be adjusted to achieve the targeted strength level. However, not all AHSS can attain their microstructure with the thermal profile of a conventional hot dipped galvanizing line with limited rapid quenching capabilities. In addition, many AHSS grades have chemistries that lead to increased surface oxides, preventing good zinc adhesion to the surface. These grades must be produced on a stand-alone Continuous Annealing Line, or CAL, without an in-line zinc pot. Continuous Annealing Lines feature a furnace with variable and rapid quenching operations that enable the thermal processing required to achieve very high-strength levels. If corrosion protection is required, these steel grades are coated on an electrogalvanizing line (EG) in a separate operation, after being processed on a CAL line.
Hot dipped galvanizing lines at different steel companies have similar processes that result in similar surfaces with respect to coefficient of friction. Surface finish and texture (and resultant frictional characteristics) are primarily due to work roll textures, based on the customer specification. Converting from one coating line to another using the same specification is usually not of major significance with respect to coefficient of friction. A more significant change in friction is observed with changes between GI and GA and EG.
Soon, we’ll release the results of a three-year study on Liquid Metal Embrittlement in resistance spot welding. It will shed light on why LME occurs, how its occurrence can be controlled, and practical preventative measures to avoid LME on the manufacturing line. Stay tuned.