News
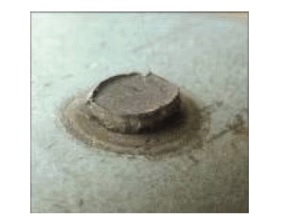
Figure 1: Full Button Nugget
Contributed by Menachem Kimchi, Ohio State University
Advance High-Strength Steels (AHSS) have been resistance welded in automotive production lines in the last few years. However, the high strength and hardness can be expected to affect spot weld failure modes during the typical peel testing and chisel testing performed for weld quality evaluation.
A well-established industry standard associated with peel testing of conventional steels is that an acceptable test is one in which the peel test “pulls a nugget” or a “full button,” as shown in Figure 1.
However, with AHSS, full button pulls are less likely due to the high Carbon Equivalent that are likely to produce hard weld nuggets. This fact is compounded by the higher yield strengths of the material that will tend to produce greater stresses concentrating at the edge of the nugget during a peel or chisel tests. Therefore, the conventional modes of testing such as peel and chisel testing may be more likely to produce interfacial or partial interfacial failure modes such as those shown in Figure 2.
With AHSS, these types of failure modes may occur even though the weld strengths may be acceptable for the intended application. Even full interfacial failures may exhibit high strength, although it may sometimes be challenging to differentiate between an interfacial failure and a “stuck” weld condition (which refers to an unfused bond of unacceptable strength).
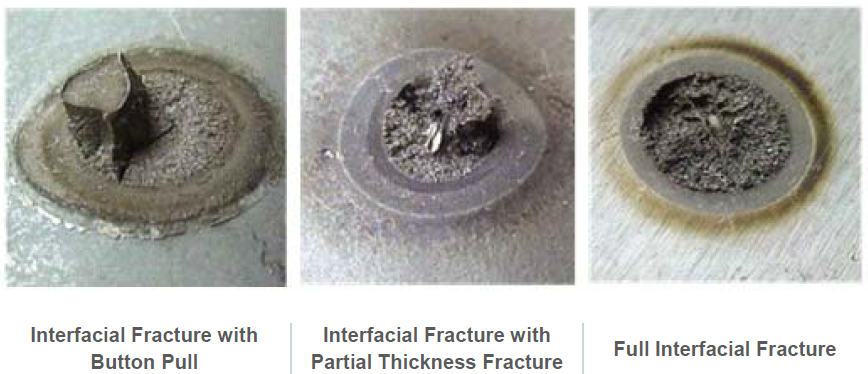
Figure 2: Failure Modes
To improve nugget failure modes with AHSS, the hard martensite must be softened. A simple and effective approach to accomplishing this is to add a temper cycle at the end of the weld (Figure 3), something that can easily be added at the end of the spot welding cycle. A sufficient amount of quench time must be included prior to tempering to allow the complete transformation to martensite, and the amount of tempering time and current will dictate how much softening occurs. Of course, the quench time adds cycle time to each weld, so production requirements mandate that this time be kept as short as possible. Depending on how hardenable the steel being welded is, other approaches that slow cooling rates may be helpful. These approaches include current pulsing, current sloping, longer weld times, and short hold times.
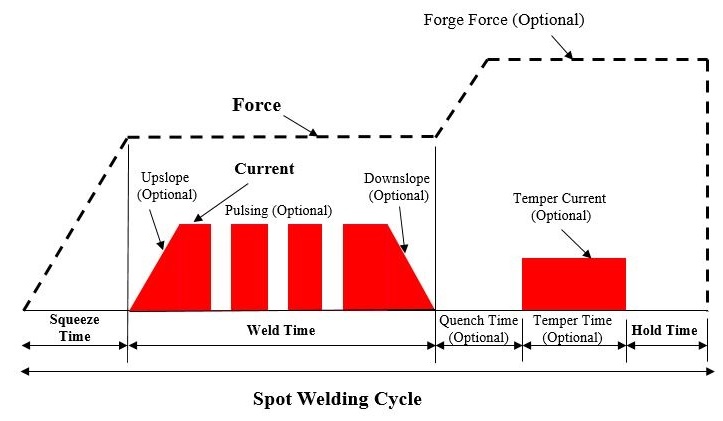
Figure 3: Spot Welding Cycle
News
The challenges to spot welding of aluminum compared to steel include a tenacious and rapidly forming oxide layer of variable thickness and composition, high electrical and thermal conductivities, small increases in resistivity with temperature, a narrow plastic range, low melting temperatures, and a high coefficient of thermal expansion. Used by permission from Menachem Kimchi,Assistant Professor-Clinical, Materials Science Engineering, Ohio State University, this excerpt from a new book by Kimchi and David Philips, Resistance Spot Welding Fundamentals and Applications for the Automotive Industry, explains these differences for those who wish a deeper understanding. This article is the first of a series by Kimchi on welding of Advanced High-Strength Steels, so stay tuned!
Thermal Conductivity and Electrical Resistivity
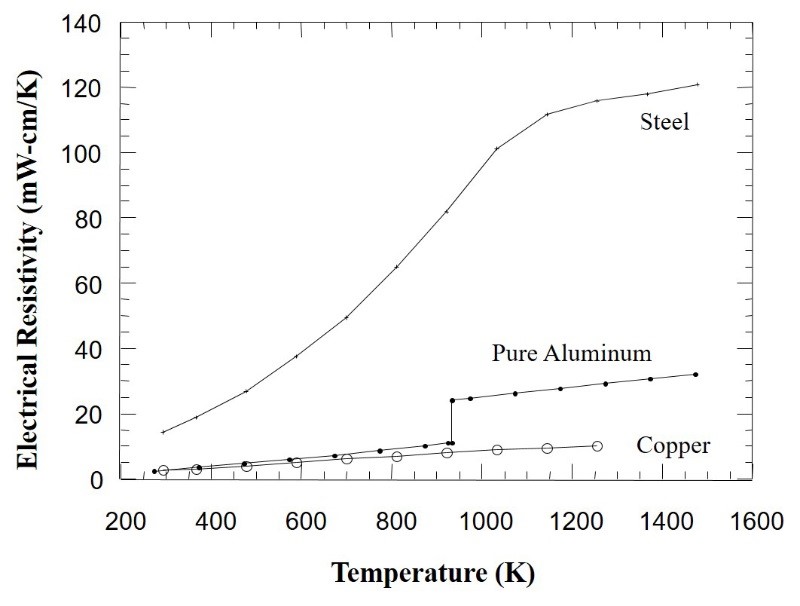
Figure 1: Electrical Resistivity of Steel and Aluminium
(compared to copper electrodes)
The Resistance Spot Welding process, one of the primary processes used in the automotive industry, works best with metal alloys such as steels that have electrical and thermal conductivities that are much lower than the copper-based electrodes used to weld them. Low electrical conductivity (or high resistivity) provides for easy I2R heating, and low thermal conductivity means heat will be extracted from the weld nugget region more slowly. The longer it takes for heat to extract, the more robust the weld. As shown in Figure 1, steel has a very high resistivity and therefore is ideal for this welding process.
Aluminum exhibits electrical and thermal conductivities that are close to copper, two additional reasons contributing to spot welding challenges with this metal. These properties dictate the need for much higher currents, and much shorter times, and therefore a less robust process. Rules of thumb regarding welding currents and times for aluminium is approximately three times the current temperature, and 1/3 of the process times for welding steel. Consequently, existing equipment cannot be used in welding aluminium because of the higher current required.
Plastic Range of Metal
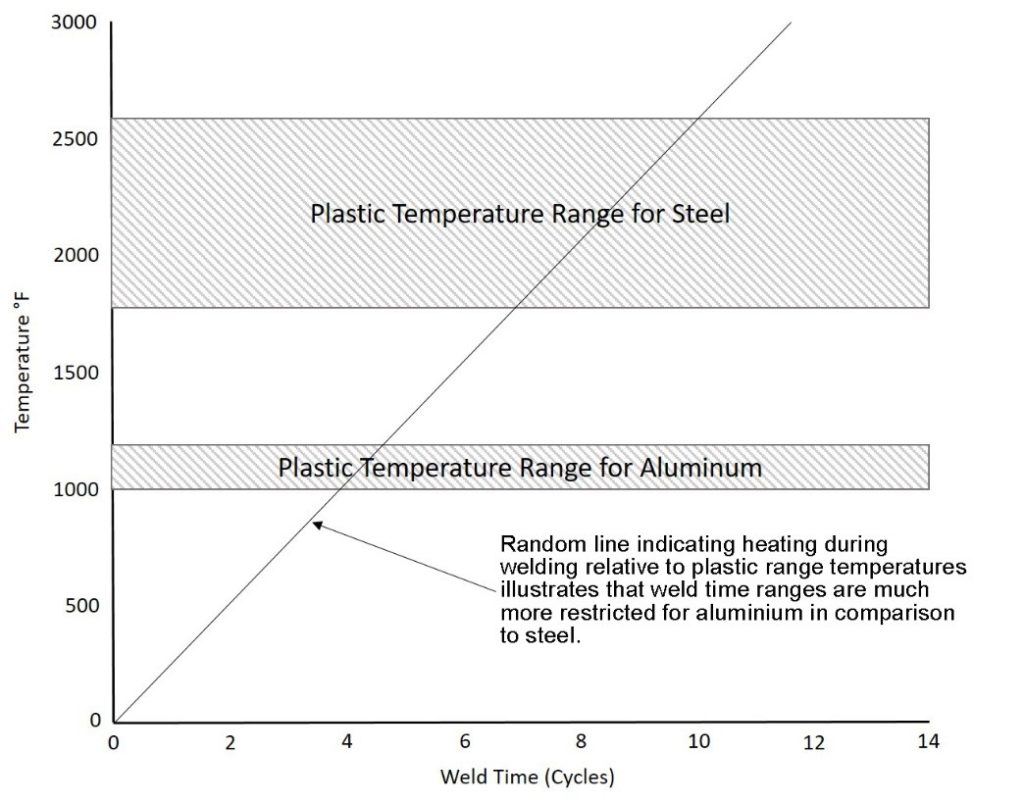
Figure 2: Typical Plastic Ranges, Steel vs. Aluminium
The plastic range of a metal can be loosely defined as the range of temperatures below its melting temperature in which the metal exhibits significant softening. The significance to spot welding is that wider plastic ranges will create a wider softened region around the weld for a longer time. This region, in conjunction with the electrode pressure, effectively “seals” the rapidly expanding (metals exhibit large volumetric expansions when they melt) molten weld nugget, and prevents it from being ejected from the weld zone (expulsion). As indicated on Figure 2 the typical plastic range of aluminum is significantly less than that of steel. The figure also includes a random heating line to illustrate the fact that a narrow plastic range not only reduces the width of the “seal” around the nugget, but also suggests that the window of welding time to produce a good weld is restricted. In summary, the narrow plastic range of aluminum combined with its low melting temperature means that the process window to create a good weld and avoid expulsion is very small.
Dynamic Resistance
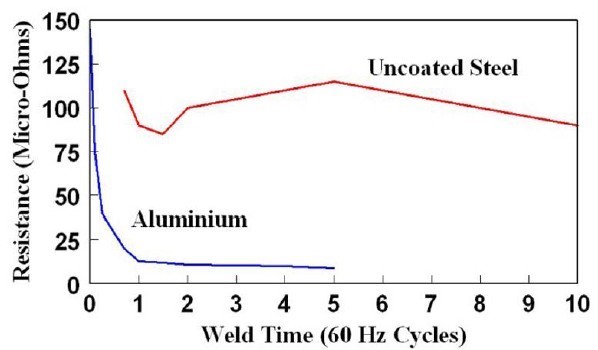
Figure 3: Dynamic Resistance Curve for
Steel Vs. Aluminium
As indicated on Figure 3, the dynamic resistance curve for aluminum is entirely different from the curve for steel. Two facts contribute to this vast difference:
1) The oxide on the surface of the aluminum, and
2) The small change in resistivity as a function of temperature.
Upon initial flow of current, resistances are extremely high due to the oxide layer which has much higher resistivity than the aluminium. This increases the likelihood of initial expulsion and will also result in significant electrode heating. The oxide layer quickly breaks down allowing current to pass more easily as resistance drops rapidly. However, as compared to the dynamic resistance curve for steel, there is no significant increase in resistance later in the cycle. The reason for this is compared to steel, aluminum’s resistivity increases only slightly with temperature as shown in Figure 3. The implication of this difference is that there is limited opportunity to grow the nugget by taking advantage of the rapid increase in resistivity, as is the case with steel.
Coefficient of Thermal Expansion
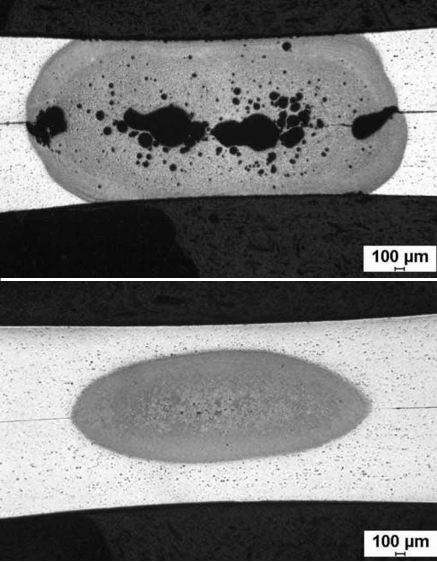
Figure 4: Aluminium Weld Discontinuities (Porosity)
Aluminum’s thermal expansion coefficient is roughly three times higher than steel. This results in greater volumetric expansion of the metal upon heating, and subsequent greater contraction upon cooling. The consequence is a greater chance not only for expulsion, but weld discontinuities such as porosity and solidification cracking (Figure 4). This may mandate the need for low inertia, fast “follow-up” weld heads which can maintain consistent force during the rapid movement of the expanding and contracting weld region. This requires more equipment and expense for the process.
Aluminium’s Oxide Layer
As discussed previously, aluminum forms an oxide layer that is tenacious and forms rapidly. The highly resistive oxide layer can be a benefit in that it significantly increases contact resistance between the sheets being welded. But maintaining a consistent oxide layer thickness is difficult since it happens naturally and rapidly as it is exposed to the environment. Because it is inconsistent, it causes inconsistencies in the weld.
On the other hand, if the oxide layer is significantly reduced by mechanical (such as grinding) or chemical (such as acid cleaning followed by a conversion treatment) methods immediately prior to welding, the need for extremely high currents will be mandated which will promote electrode sticking and accelerated wear.