Manufacturers embrace Advanced High Strength Steels as a cost-effective way to satisfy functional and regulatory requirements. The following are just a few examples where automakers have attributed improved performance and lightweighting due to the use of these advanced steels.
KIA EV9
The Kia EV9, Kia’s first three-row electric flagship SUV, is based on the Electric Global Modular Platform (E-GMP).K-59 Kia EV9 won the 2024 North American Utility Vehicle of the Year™ (NACTOY) AwardK-60, and was named a 2024 Top Safety Pick by IIHS, the Insurance Institute for Highway Safety.K-61 Kia deployed hot stamped parts in the passenger safety cage for enhanced passenger protection and crash energy management.K-62
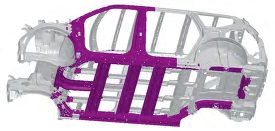
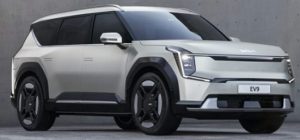
Figure 1: Hot stamped parts increase the average tensile strength in the 2024 Kia EV9K-62
Tesla Cybertruck and Model Y
Much press has been given to the “ultra-hard stainless steel” used on the Cybertruck skin panelsT-46, but there are several high strength and advanced high strength steel parts on the vehicle as well. According to the Cybertruck Collision Repair Manual,T-47 Tesla defines mild steel as having a tensile strength less than 270 MPa. The tensile strength of high strength steels ranges from 300 MPa to 700 MPa. Ultra high strength steels are those with a tensile strength greater than 800 MPa. Figure 2 presents a breakdown of materials used in the body structure.
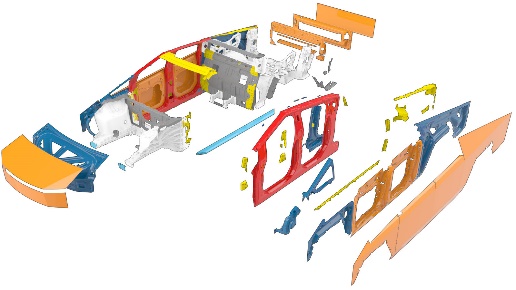
Figure 2: Cybertruck Body Materials. Dark blue = mild steel; yellow = high strength steel; red = ultra high strength steel; orange = stainless steel.T-47
A video by Munro Live with Lars Moravy, Tesla’s Head of Vehicle Engineering, shows that the Cybertruck body side inner is formed from a laser welded press hardened steel.M-65-2 An interview with Thomas Ausmann, former global advanced manufacturing technical advisor at Tesla, confirms that Tesla hot stamps the double-door rings, which represents the first hot-stamped part that Tesla had ever produced internally at any of its plants.B-78 Figure 3 shows a Cybertruck hot-stamped body side inner.
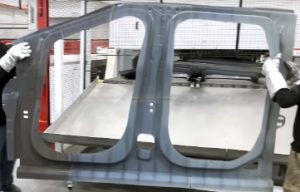
Figure 3: The Cybertruck double door ring made from a laser welded blank is the first hot stamped part that Tesla ever produced internally at any of its plants M-65-2 B-78
The Model Y Collision Repair Procedures ManualT-48 highlights that there are several ultra high strength steel parts in the body structure. Another video from Munro LiveM-70 confirms that the ultra high strength steel in the body side aperture is press hardened, hot stamped steel. Simwon NA is the likely supplier of these hot stamped parts.Y-15
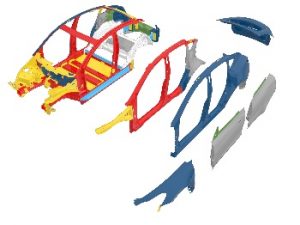
Figure 4: Press Hardened Hot Stamped Steel in the Model Y Body Side Outers and Inners. Dark blue = mild steel; yellow = high strength steel; red = ultra high strength steelT-48
Li Auto L8
The Li L8 is a luxury range-extended battery electric SUV equipped with an autonomous driving system produced by Chinese manufacturer Li Auto. Hot-formed steel is used in safety-critical areas such as the A-pillar, B-pillar, C-pillar, door sills, and door intrusion beams, accounting for 28.9% of the entire body-in-white, with high strength steels accounting for over 75% of the body structure. Hot-formed steel parts are shown in red in Figure 5, with ultra high strength steel shown in yellow, high strength steel shown in dark gray, and mild steel parts colored in blue.X-2
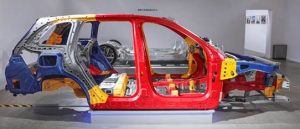
Figure 5: Nearly 30% of the Li Auto L8 body-in-white is made from Hot Stamped Press Hardened Steels.X-2
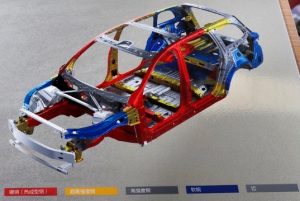
Honda Civic
The 2025 Honda Civic Hybrid, based on the 11th Generation Honda Civic platform launched for the 2022 model year, uses high strength and advanced high strength steel throughout their Next-Generation Advanced Compatibility Engineering™ (ACE™) body structure. Honda defines high-strength steel (HSS) as any steel with a tensile strength of 340 MPa or higher. Ultra-high-strength steels (UHSS) are those with a tensile strength of 980 MPa or higher.H-68
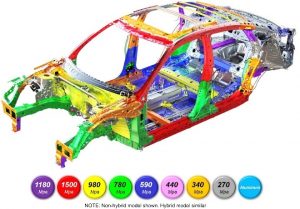
Figure 6: The body construction of the 2025 Honda Civic uses high-strength steel and advanced high-strength steel for enhanced passenger protection.H-67
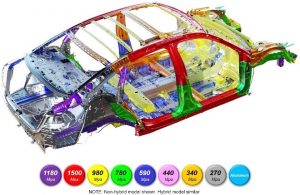
Nissan Rogue
The 3rd Generation Nissan Rogue, launched for the 2021 Model Year, makes extensive use of advanced high strength steels, including 3rd Gen AHSS.
Nissan deploys AHSS grades for 35% of the body structure, an increase of more than 10% compared to the prior version.L-67 Hot stamped press hardened steels, not used in the prior model, helps this Nissan Rogue achieve improved safety, fuel efficiency, and customer satisfaction. Figure 7 shows how various steel grades are deployed in the body structure.
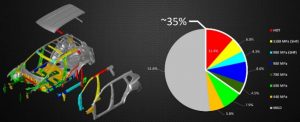
Figure 7: Nissan Rogue Body-in-White Uses Press Hardened Steels and 3rd Generation Advanced High Strength Steel Grades.L-67
The Rogue’s B-pillar is cold stamped from a tailor welded blank of super high formable 980 (SHF 980) and super high formable 1180 (SHF 1180) steel, allowing Nissan to realize the same benefits of hot stamping at a much higher productivity, as highlighted in Figure 8 L-67. Both of these super high formable grades can be considered 3rd Generation Advanced High Strength Steels. (See the information on the 2018 Infinity QX50 SUV here) .
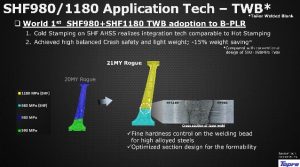
Figure 8: The Nissan Rogue uses a laser welded blank formed from two 3rd Generation Advanced High Strength Steels. L-67
A critical enabling technology in the use of SHF 980 and SHF 1180 is the development of design guidelines for welding stacks that include those materials. These guidelines use weld gun control and panel positioning to prevent unneeded additional tensile stress in the weld stack.L-66 Minimizing the tensile stress in the weld stack helps address the risk of liquid metal embrittlement as does extending the hold time portion of the spot weld cycle in order to lower the temperature prior to releasing the electrodes.L-67
Chevrolet Blazer EV
The Chevrolet Blazer EV is built on the same architecture as the Chevrolet Equinox EV, Cadillac Lyriq, Honda Prologue, Acura ZDX EV, among others.E-14
Fifteen percent of the body structure are ultra high strength steels, including multiphase, martensitic, and 3rd Generation Steels having a tensile strength of at least 980 MPa. An additional 11% are stamped from press hardened steels. The breakdown of the Blazer EV body structure is shown in Figure 9.
Regarding the battery pack, part of the General Motors battery management system known as the Rechargeable Energy Storage System (RESS), 43% of the all-steel construction is made from grades with tensile strength of at least 980 MPa. (Figure 10).
Instead of using press hardening steels for the B-pillar, General Motors stated that there was a cost savings in addition to a mass savings by using 3rd Generation AHSS in this application. This required development of a material grade specification capable of use globally, along with forming and welding practices for robust production. (Figure 11).
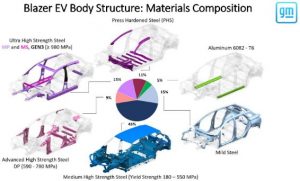
Figure 9: 35% of the Chevrolet Blazer EV body structure is made from Advanced High Strength Steels with a tensile strength of at least 590 MPa. E-14
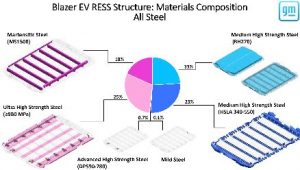
Figure 10: 43% of the Chevrolet Blazer EV Rechargeable Energy Storage System structure is made from Advanced High Strength Steels with a tensile strength of at least 980 MPa. E-14
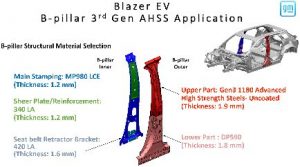
Figure 11: Use of 3rd Generation Advanced High Strength Steels in the B-Pillar of the Chevrolet Blazer EV led to cost savings and mass savings while maintaining crash and safety performance. E-14
Steel, and specifically advanced high strength steel, satisfies automotive industry requirements for safety, emissions, fuel efficiency, manufacturability, durability, and affordability.
Affordability
The automotive industry has adopted light-weighting as a key part of their greenhouse gas reduction strategy. This strategy, however, must be executed in an affordable manner.
Key reasons to deploy advanced high strength steels in automotive body structures include better performance in crash energy management and increased strength allowing this performance to be achieved with thinner materials, translating into lower vehicle weight. Thinner gauges mean a reduced amount of purchased material is needed to achieve this enhanced performance.
Today’s steels enable significant mass reduction, while meeting crash and other functional requirements, while preserving affordability. WorldAutoSteel vehicle programs and competitive benchmarking studies (here, here and here) have clearly demonstrated that optimized steel body structures can be constructed with little to no increases in total system cost relative to conventional body structures.
Recognizing these benefits, global automakers continue to base their fleet on steel-intensive body structures and rely on advanced high strength steel to help them meet customer expectations and government regulations in the most cost-effective way possible.
Most steel companies are extending their research and development efforts to expand the range of properties available through these new steels, to enable the production of safe and environmentally friendly vehicles.
Safety
The percentage of high strength steels used in light vehicles relative to other materials continues to grow, and much of the growth has been fueled by increasingly stringent safety regulations and ratings systems. Consumers are demanding safe cars, and governments are responding with new tests and standards that influence auto body structures, design and materials. The results of vehicle safety performance tests are therefore a strong incentive affecting consumer purchasing decisions.
In the United States, the National Highway Traffic Safety Administration (NHTSA) sets standards for vehicle safety, such as those for impact resistance, restraints, and fuel economy.N-26 Testing by the U.S. Insurance Institute for Highway Safety (IIHS) has encouraged improved frontal, side, and rear impact ratings, as well as roof strength and rollover ratings, for vehicles on the road today.I-5 Numerous New Car Assessment Programs around the world measures vehicle performance in a variety of crash tests, including front, side and pole impacts, and impacts with pedestrians with the goal of informing the public about the relative safety performance amongst vehicles. A limited number of these are shown in Citations G-5, E-13, and J-25. Citations C-35 and C-36 summarize the testing that goes into these global New Car Assessment Program standards. Meeting these standards and improving performance often requires the addition of weight to the vehicle.
Diligent application of advanced steels leads to safe, lighter-weight body structures. Tailoring the engineering design and forming approaches to the material characteristics and properties allows for optimized use of these newer grades and avoids excess or redundant mass in the structure. The FSV program showed that natural, non-linear load paths allow for more effective crash management and lighter-weight structures, attainable through design optimization and rigorous application of AHSS.
Deploying Advanced Steels in Automotive Body Structures
Several key considerations drive material selection for automotive applications, including safety, fuel efficiency, environmental performance, manufacturability, durability, and quality. For exposed parts, aesthetic concerns related to paint finish and dent resistance are also important. These factors manifest themselves differently in each component of the vehicle, and materials are selected to match each set of performance requirements in the most efficient means possible.
Crash Performance
Perhaps the most critical design considerations for a vehicle structure relate to its ability to carry the required static and dynamic loads, particularly during a crash. Both materials strategy and geometric design play important roles in determining the final load paths and part details.
Two generalized areas of the car have very different safety requirements, as shown in Figure 1. The passenger compartment, enclosed in a rigid “safety cage,” is designed to protect the passengers in the event of a low or high-speed crash; the structure should prevent any deformation or intrusions that would compromise the integrity of the cage structure and impinge on the space around the passengers.
“Crumple zones,” located at the front and rear of the vehicle, are designed to absorb as much energy as possible in the event of a front or rear collision. By absorbing the energy over a distance, the crumple zone will cushion the impact and help preserve the structural integrity of the passenger compartment.
Table 1 presents general guidelines for materials selection in the crumple zone and the passenger compartment.
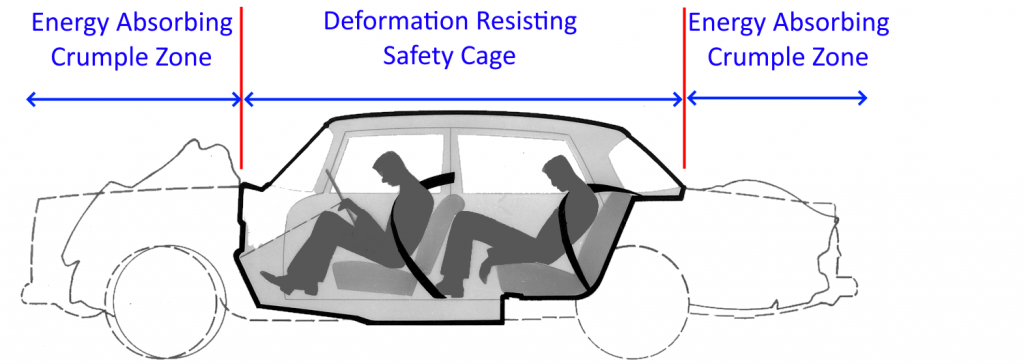
Figure 1: The major crash management zones of a vehicle.
Table 1: Steel Grade Strategies For Targeted Safety Performance
Crash Zone |
Performance Requirements During Crash |
Material properties to meet need |
Evidence of Performance |
Potential Steel Selection |
Energy Absorbing Crumple Zone |
High energy absorption over a distance |
High work hardening, strength, and ductility |
Large area under the stress-strain curve |
Dual Phase,
Complex Phase,
Transformation-Induced Plasticity,
3rd Generation Steels |
Deformation Resisting Safety Cage |
No deformation or intrusion |
High yield strength |
Highest tensile strength |
Martensite,
Press Hardening
Hot Formed,
>980 MPa Dual Phase,
>980 MPa Complex Phase |
Automotive components are designed so that together they form a structure that meets all crash, safety, and functionality requirements, including those enforced by regional regulatory bodies and those set internally by car companies. Steel grade characteristics and properties guides the selection for specific applications, as shown schematically in Figure 2.C-5
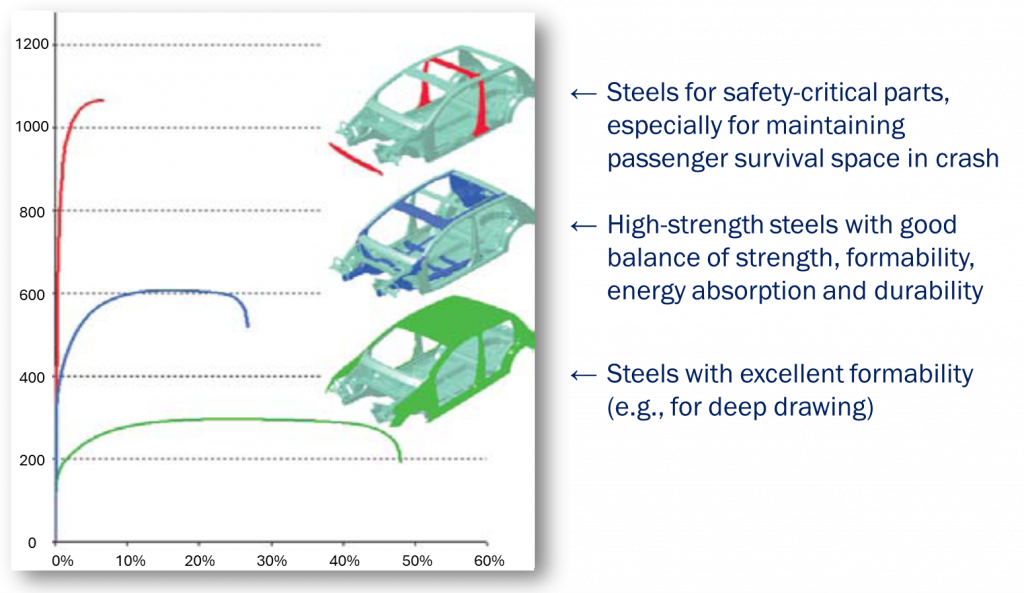
Figure 2: Comparison of stress-strain curves for different applications with body structures.C-5
Stiffness
Counter to the opinion of many, stiffness is not a function of a material’s strength. It is, however, a function of part geometry, elastic modulus and thickness. As an example of how part geometry influences stiffness, consider a sheet of paper. Stiffness improves dramatically once a crease is folded into it, yet the strength has not changed.
In automotive bodies, stiffness is related to handling and safety, as well as noise, vibration, and harshness characteristics.
A common strategy is to deploy AHSS for increased strength while decreasing weight by using thinner material. Casually applied, stiffness can suffer as a result. Geometry, specifically the moment of inertia of the cross-section about the primary load axis, plays a significant role in determining stiffness. The flexibility to adjust cross sectional and overall geometries allows for structural design solutions that more efficiently carry loads in the vehicle. The use of AHSS offers many advantages in this process because high work hardening rates increase formability, allowing for improved shapes for optimal efficiency. Additionally, AHSS typically possess high bake-hardening ability which can improve the final strength of a component after forming and paint-baking (curing).
Many lower-density metals like aluminium also have a lower elastic modulus than steels. To have an equally stiff component as one made of steel, the component from the lower-density material must have a combination of increased thickness or increased geometry. That increased thickness negates some of the perceived weight savings from the alternate material. Furthermore, lower-density materials must have sufficient ductility to be able to withstand the forming of the additional geometric features like in-part darts and beads and deeper draws associated with the increased shape required for stiffness improvement.
Forming and Manufacturability
Advanced high strength steels were developed partly to address the decreased formability typically associated with increased strength in conventional steels. AHSS grades are available at higher strength levels than conventional high strength steels, and have high work hardening and bake hardening characteristics that allow for increased formability and opportunities for additional optimization of part geometries. Both overall elongation and local elongation properties are important for formability, and global steelmakers have developed grades to address these needs.
Blog, main-blog, News
As robotaxi companies in the USA prepare to launch their autonomous vehicles in more cities, safety is in the spotlight again. And rightly so. Autonomous vehicle safety challenges must be addressed and with the Steel E-Motive Level 5 autonomous concept, we did that.
Many autonomous mobility service companies have relied on two factors when developing their vehicles: active safety systems which help the vehicle avoid or mitigate the extent of a crash, and a maximum vehicle speed limit, which will reduce the extent of injuries to the occupants.
But the fact is that these vehicles are going to be out in mixed-mode traffic situations. There will be accidents – however much we all attempt to do everything possible to avoid them. When we developed the Steel E-Motive (SEM) body structure concepts for fully autonomous ride-sharing electric vehicles, we agreed on two basic principles – that these vehicles would operate at high speeds (top speed of 130 kph) in mixed mode traffic conditions, and that we, therefore, needed to engineer passive safety structures that met global high-speed crash requirements to protect occupants and the battery system in these use-case conditions. In this process, we discovered that no other provider of autonomous ride-sharing electric vehicles had fully shared details of passive safety structures engineered to those same high-speed crash standards. Autonomous vehicle safety had been addressed only on a limited basis.
Fortunately, our vehicle design process benefitted from a massive portfolio of modern advanced high-strength steels (AHSS) available through member companies of WorldAutoSteel. Steel E-Motive (SEM) was developed to show how AHSS can enable sustainable, comfortable, economical, and safe ride-sharing vehicles by 2030.
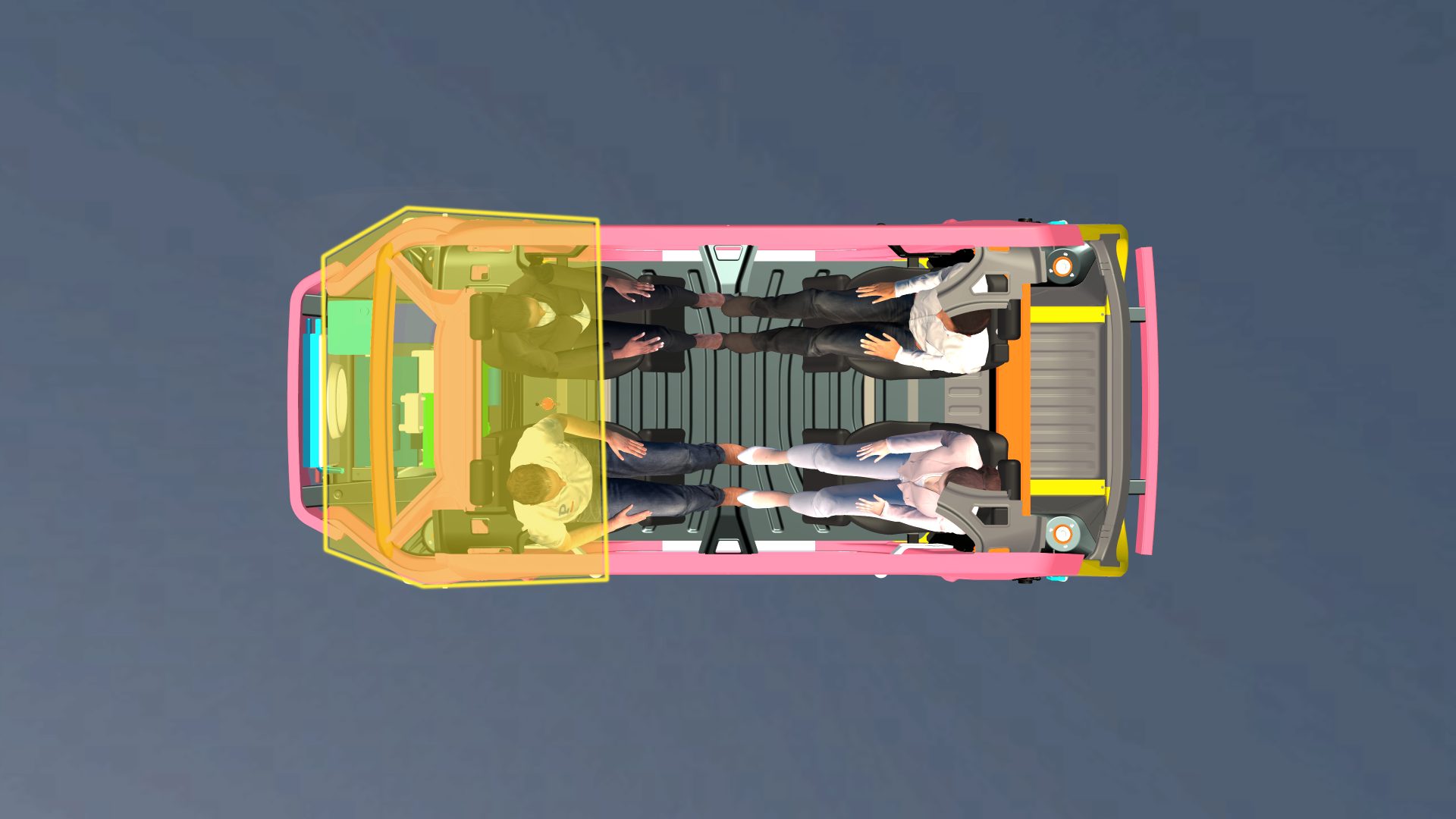
The AHSS Extended Passenger Protection Zone provides excellent cabin intrusion protection and ultimately lower risk of injury. PHS provides formability for challenging geometries, and Martensitic steel (MS) provides the strength to limit intrusion.
Visit this link to download the full engineering report: Steel E-Motive
The result is one of the first robotaxis to fully detail and report compliance to global high-speed safety standards. In developing Steel E-Motive, we targeted conformity with seven US crash standards, including US NCAP (New Car Assessment Program) IIHS and FMVSS (Federal Motor Vehicle Safety Standards) front, side, and rear impact tests while also assessing performance against worldwide protocols, including NHTSA (US) Euro NCAP (European) and China’s GB 38031 standard for battery protection.
As an example, Steel E-Motive achieves the highest IIHS rating of “good.” This is particularly important as IIHS (the US-based Insurance Institute for Highway Safety) is highly regarded for its dedication to reducing deaths, injuries, and property damage from motor vehicle crashes.
Most production vehicles use new generation, advanced high-strength steels, and technologies. We had no fewer than 64 AHSS materials to select from, enabling us to choose exactly the right steel for every need and purpose in the vehicle, including safety protection. These make a car stronger, more fuel efficient, and safer.
Nearly all vehicles on the road today are made of steel because it has the broadest range of properties while being the most affordable structural material for designing safe vehicles. Steel has a unique capacity to absorb an impact, and, therefore, to diffuse crash energy. It also becomes harder when it’s crushed, which means it will become stronger on impact, retarding further penetration into the vehicle’s passenger zone.
Taking on Autonomous Vehicle Safety Challenges
Here is an outline of how we designed steel’s benefits into the Steel E-Motive (SEM) concept when considering front and side crashes.
SEM features a high-strength front protection zone, which reacts to the crush loads and minimizes intrusion for the occupants in front crashes. The crush zones have been engineered to decelerate the vehicle progressively. The longitudinal mid rails, featuring tailor-welded blanks fabricated with Dual Phase steels, are tuned to give a lower deceleration pulse into the passenger cabin to minimize injury threat. The side crush rails are designed to minimize intrusion into the cabin for occupant protection. Finally, a novel design geometry and Press Hardened Steels enable the new glance beam architecture to force the vehicle off of the Small Offset barrier; the resulting “glance-off” achieves significantly reduced crash energy and pulse into the passenger cabin.
When considering side crashes, we engineered the body structure for the IIHS 60kph deformable barrier test and 30kph side pole test, assessing both occupant and battery protection and achieving the IIHS “good” performance rating. Our side structures are comprised of a large one-piece tailor-welded door ring fabricated with press hardened steels, the TRIP steel B pillar housed in the side scissor doors, and a roll-formed hexagon rocker beam fabricated with Dual Phase steel.
These attain a very safe design, giving good levels of protection for both the occupants and battery modules, exceeding 30mm intrusion clearance at critical measurement points.
SEM was also engineered for rear crash and roof crush, and once again, the robust steel-intensive architecture exceeds crash standard requirements.
Electric-powered vehicle sales are accelerating, reflecting industry investment, and will soon achieve market domination from the combustion engine. In megacities, where congestion, pollution, and exorbitant vehicle ownership costs reign, Autonomous cars will replace drivers, and ride-sharing will become the norm. As we look into the future and recognize the need for these vehicles to offer comfortable, safe, affordable, and sustainable transportation, we will still be designing them by harnessing the unique properties of steel.
Visit this link to download the full engineering report: Steel E-Motive