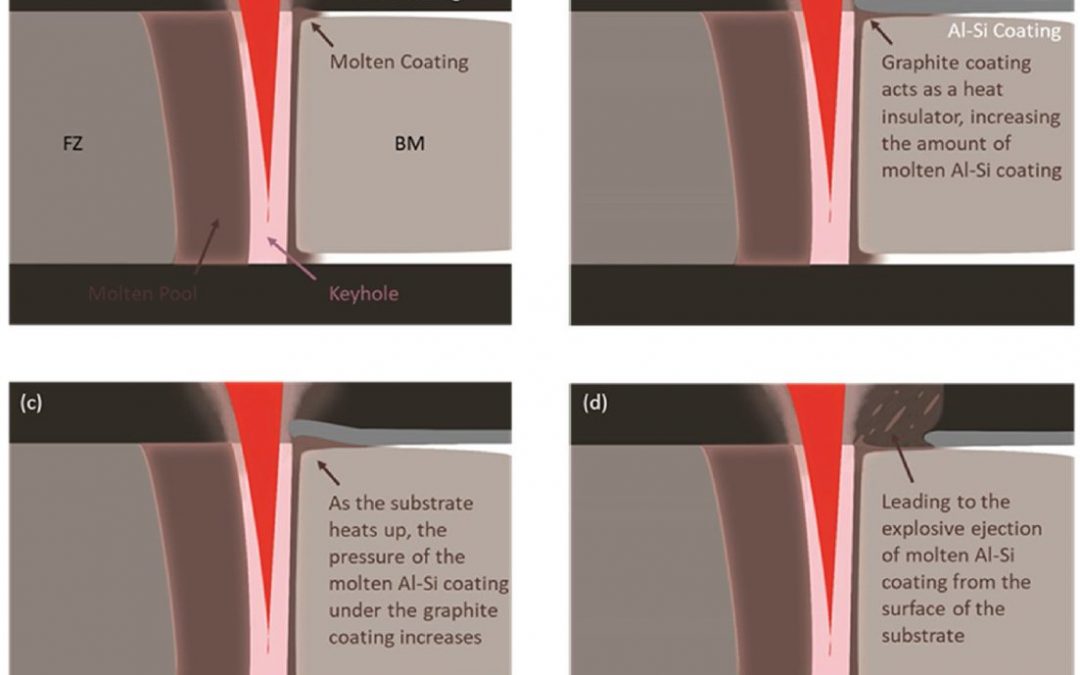
Microstructural Effects of Adding Colloidal Graphite to Al-Si-Coated PHS
Optimizing weld morphology and mechanical properties of
laser welded Al-Si coated 22MnB5 by surface application of
colloidal graphite
Researchers at University of Waterloo discovered the microstructural effects of adding colloidal graphite to Al-Si coated 22MnB5 Press Hardened Steel.K-51 Laser welds were made on 1.5 mm thick Al-Si coated 22MnB5 PHS perpendicular to the rolling direction. Pure colloidal graphite suspended in isopropanol base was applied to the area being welded and the resulting graphite coating after evaporation ranged from 5 µm to 130 μm for testing. Parameters used for the weld are: 4kW power, 6m/min welding speed, beam diameter of 0.3 mm, and laser defocus of 6mm. Samples were then hot stamped by heating for 6 min to 930 ᵒC in a furnace and then water quenched at a cooling rate greater than 30 ᵒC/s.
Al-Si coating is excellent at preventing oxidation and decarburization of high strength steel at elevated temperatures. However, during welding there is diffusion of Al into the fusion zone which stabilizes ferrite at elevated temperature reducing the strength of the welded joint. Colloidal graphite coating decreases the Al content and increases C content of the fusion zone. As shown in Figure 1, The mechanism for reduction in Al content is due the graphite coating acting as an insulator to the Al-Si coating which then causes an ejection of the molten Al-Si coating from the surface. Figure 2 displays a proportional reduction of Al in the fusion zone with increasing graphite coating thickness up to 40 μm where after the reduction in Al is minimal. This is attributed to the initial reduction of Al being caused by the ejection of the molten Al-Si from underneath the graphite coating. Graphite coating greater than 40 µm does not aid in additional ejection of Al-Si and the Al-Si coating already diluted in the weld pool will not be removed.
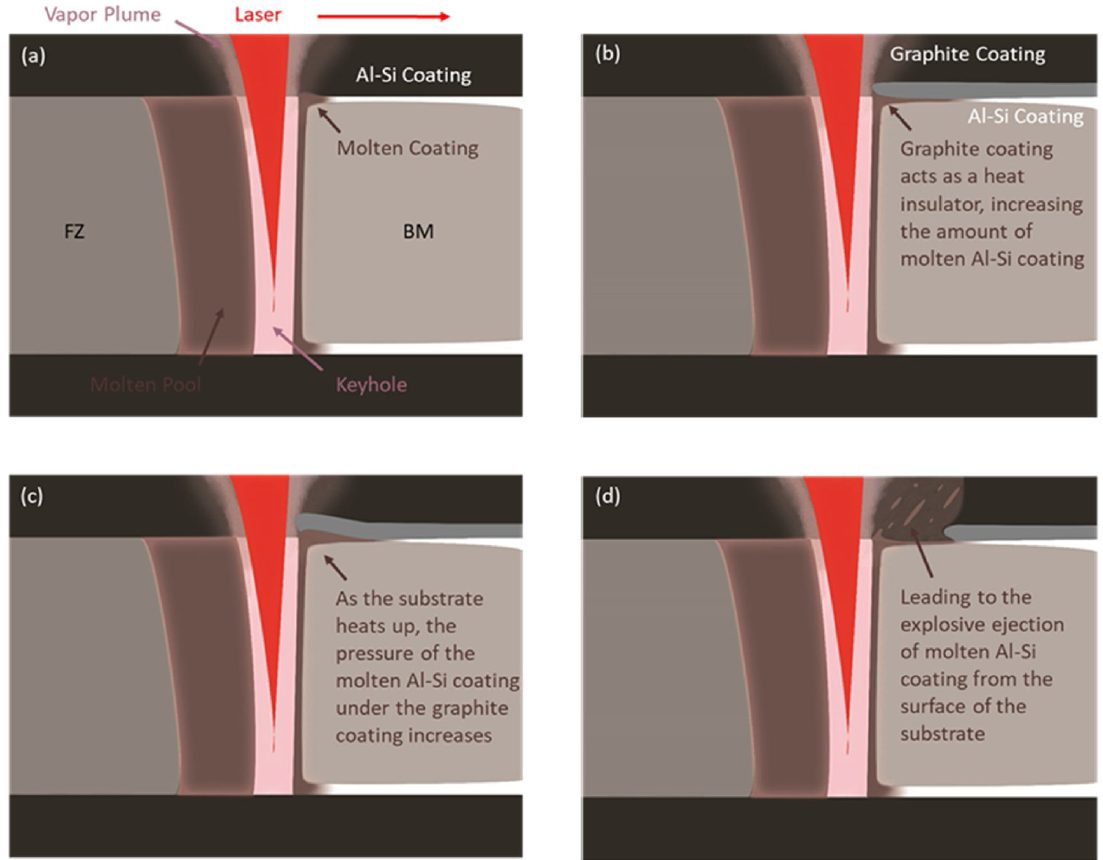
Figure 1: Al-Si ejection mechanism.K-51
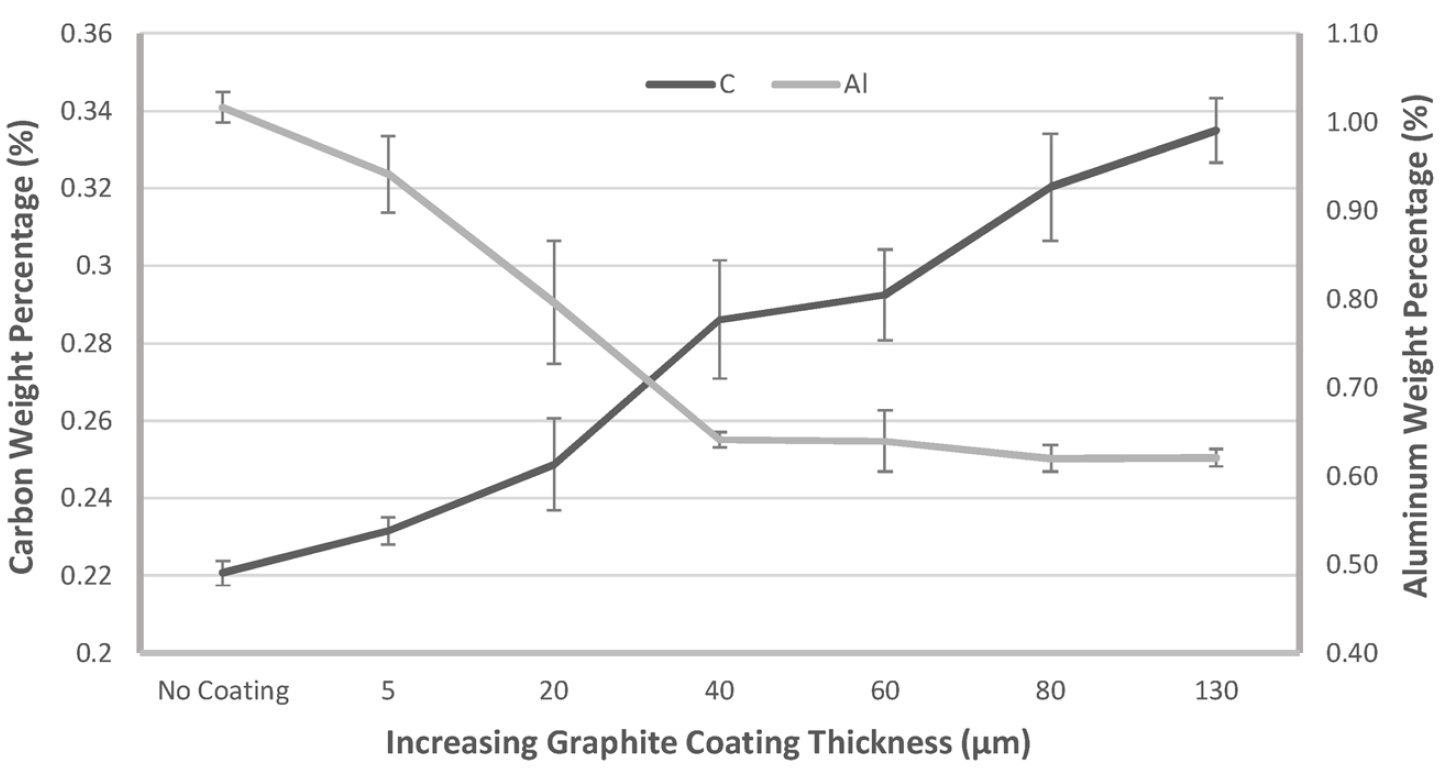
Figure 2: Al and C content in weld with increasing graphite thickness.K-51
Summary
Ferrite concentration in the fusion zone was reduced from approx. 40% with no graphite coating to approx. 2% with 130 μm graphite coating thickness (Figure 3). The increase in C content and reduction in Al content resulted in an increase in austenite being stabilized at elevated temperature rather than ferrite and therefore a larger percentage of martensite results after hot stamping. The average fusion zone hardness increased from 320 HV with no coating to 540 HV with 130 μm coating thickness (Figure 4). The weld strength of the sample with no graphite coating was 1249±15MPa whereas the weld strength with a coating of 130 µm was 1561±7MPa which matches the base metal (Figure 5). With an increase in graphite coating thickness there is an increase in weld strength that can eventually match the base metal strength.
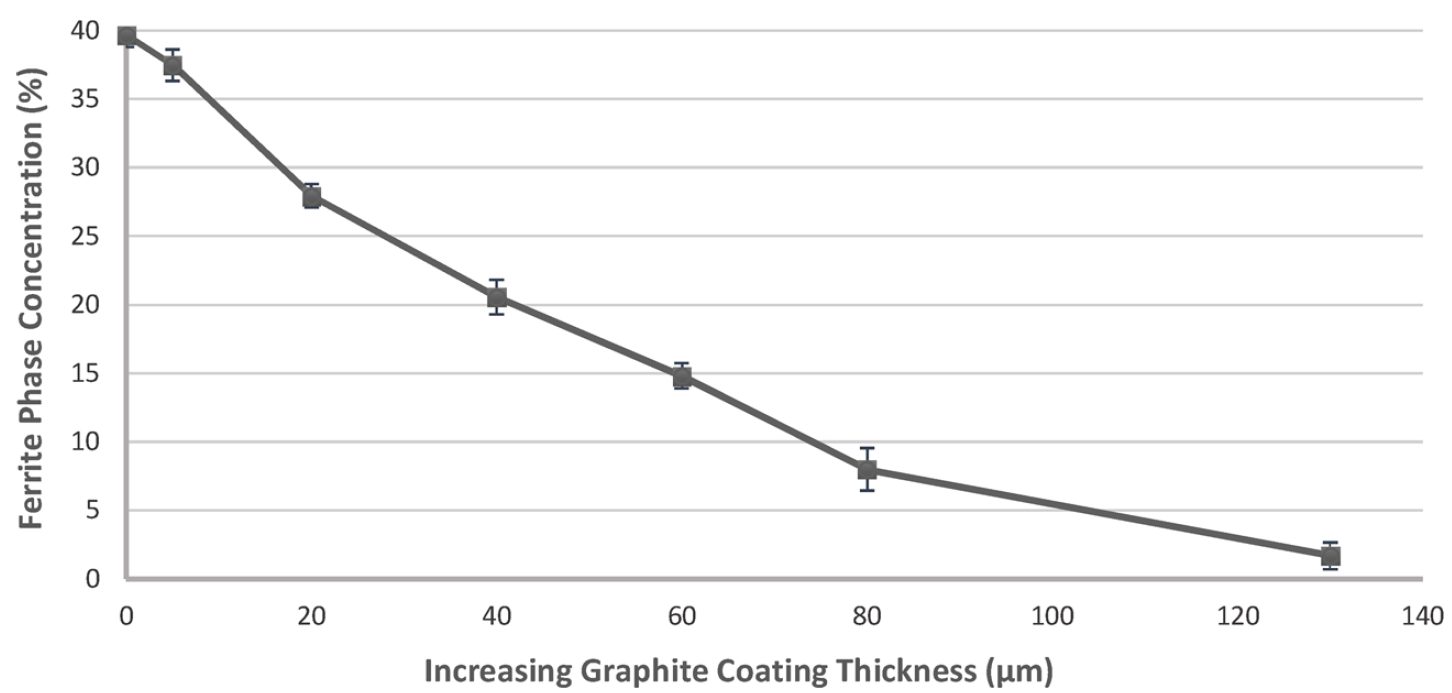
Figure 3: Ferrite concentration in weld.K-51
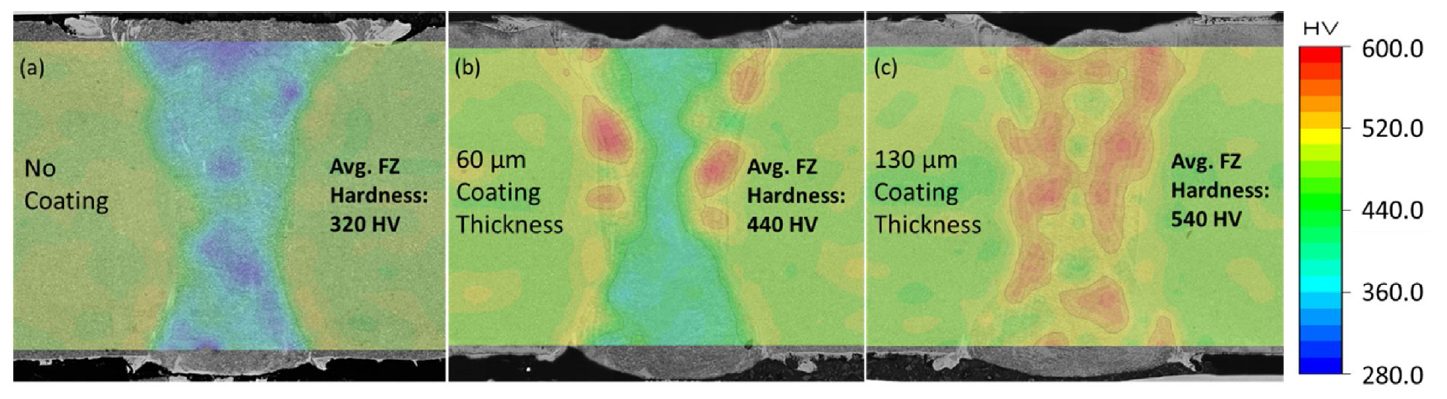
Figure 4: Fusion Zone Hardness vs. Graphite Thickness.K-51
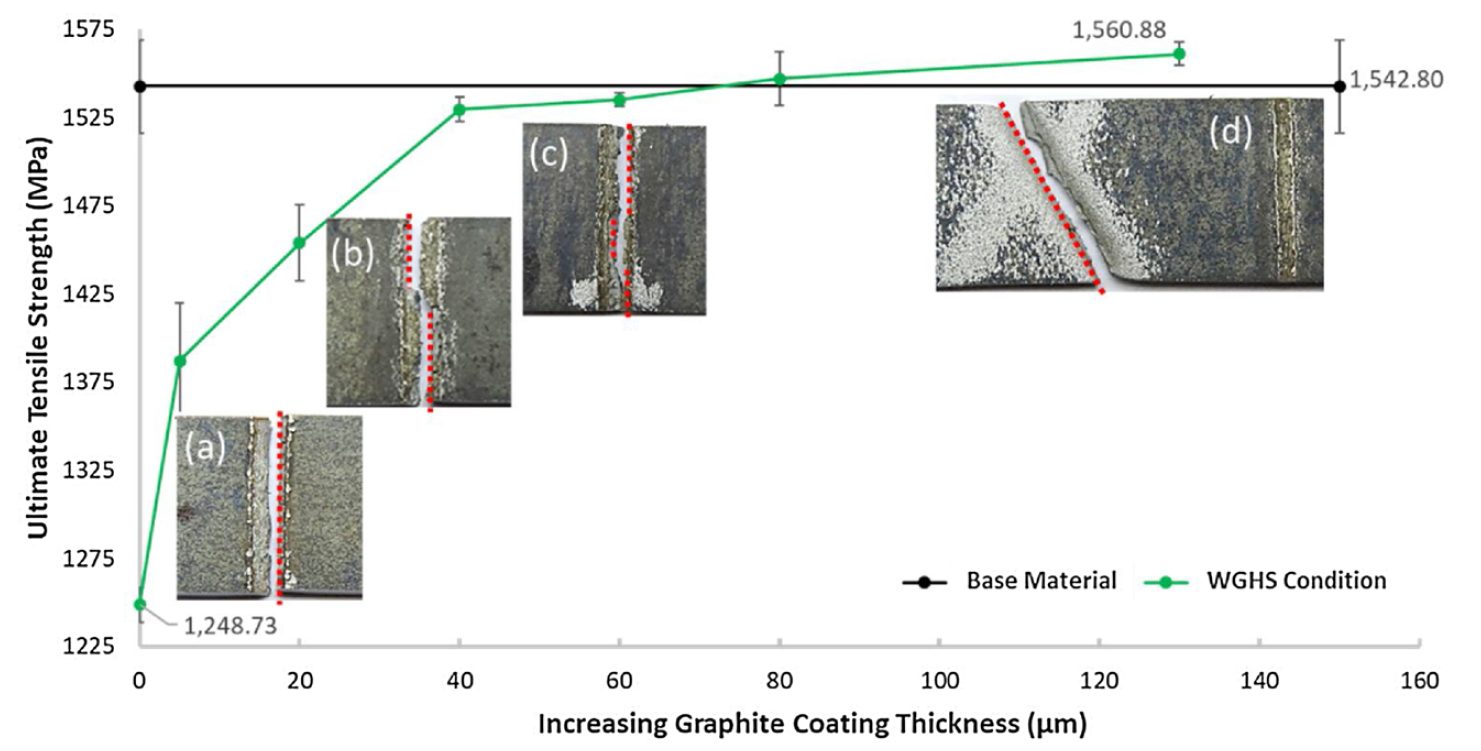
Figure 5: Weld strength vs. Graphite Thickness.K-51