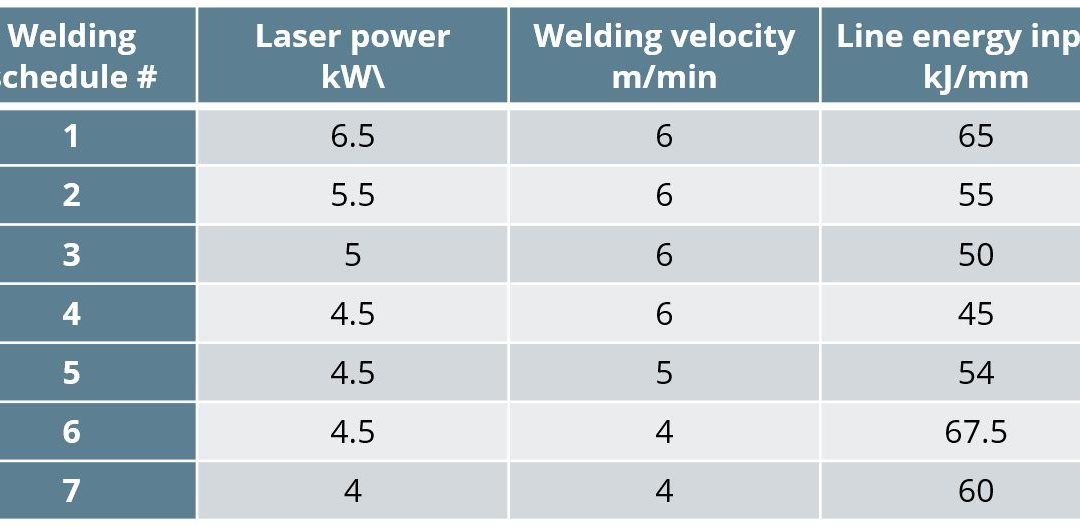
Formation mechanism of LME in laser lap welding of zinc-coated 3rd Gen steels
In this study by Shanghai Jiao Tong University and General Motors Company on liquid metal Embrittlement (LME)Z-11, hot dipped 1.2mm galvanized QP980 steel sheets with a 6 micron thick zinc rich coating on top of a 2 micron thick transition zone to the base metal were used. The laser welder is tilted 5º ahead of weld pool to avoid back reflection. The welding velocity was varied from 4-6 m/min and laser power from 4-6.5 kW. Referencing Table 1, Schedules #1–4 were intended to investigate effects of laser power on LME cracks using 6 m/min. schedules #4–6 varied velocity with the laser power staying constant at 4.5 kW. In addition, schedules #6 and #7 varied laser power at a relatively lower welding velocity of 4 m/min.
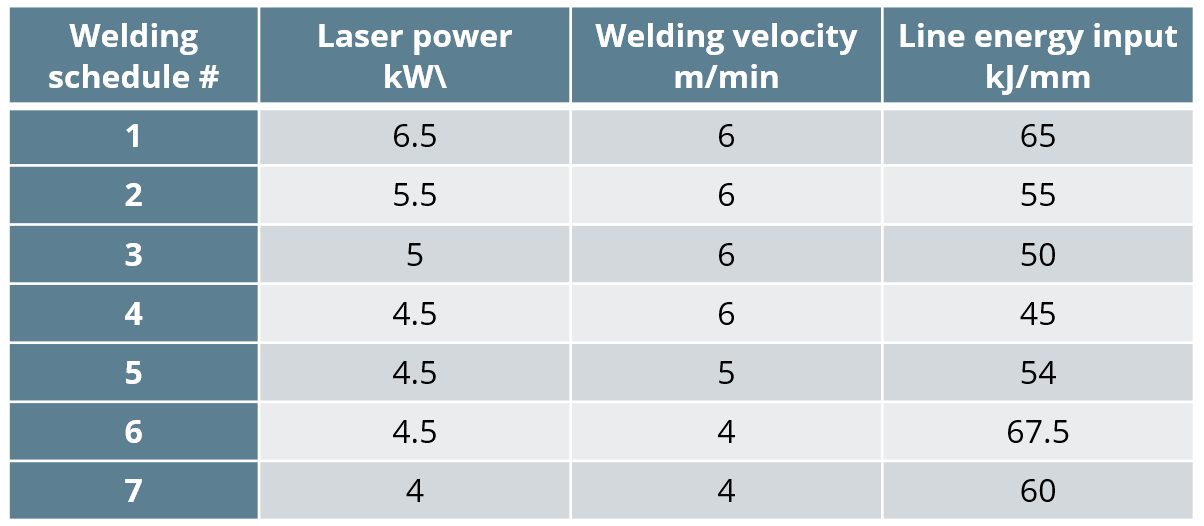
Table 1: Process Parameters.Z-11
Full penetration welds did not result in cracking while partial penetration welds with lower energy density did. The lowest energy density welds resulted in no cracking but the penetration was barely beyond the faying surface. Cracks resembles an inverse “Y” where the cracks initiated at the faying surface and propagated along the direction of solidification towards the weld centerline. Cracks at notch root were wider than centerline crack indicating grain boundary separation. The fracture method is characteristic of quasi-cleavage indicating that the grain boundaries were embrittled by Fe-Zn intermetallics. Crack initiation at the faying surface can be explained by the residual stresses that form highest at the faying surface during cooling. This stress concentration is eliminated with full penetration weld pass as well as in wide and shallow passes as seen in Figure 1.
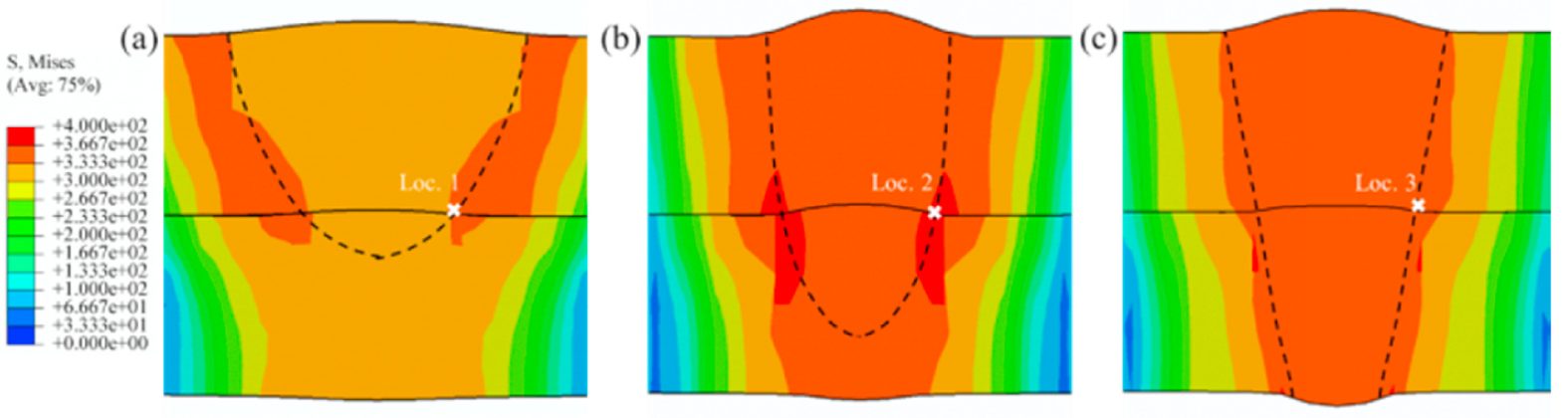
Figure 1: Von Mises Stress Distribution.Z-11
Because the process of laser welding is so short, the vaporized zinc and intermetallics do not have time to outgas completely. The liquid zinc diffuses along the austenite grain boundaries and stabilize ferrite given ferrite has a higher solubility of Zn compared to austenite. The remaining liquid Zn reacts with the ferrite to form the solid Γ-Fe3Zn10 which is a hard brittle intermetallic that can easily induce cracking. Another peritectic reaction occurs with Γ-Fe3Zn10 and liquid zinc forming another brittle intermetallic δ-FeZn10. The stabilized ferrite and intermetallics occur before austenite transform to martensite and remains even after this reaction occurs.
The formation of the intermetallics between the grain boundaries decreases ductility and with the combination of the high residual stress and stress concentration at the faying surface, the crack initiates and propagates. The cracking follows the grain growth to the center of the weld. Liquid Zn accumulates at the center of the weld and results in Fe-Zn intermetallics with low ductility that can eventually propagate cracking that occurs at the edge of the weld. Welds that result in a smaller cross-sectional area on the faying surface benefit from the reduction in vaporized Zn that cannot be outgassed while full penetration welds with keyhole mode can allow Zn to out gas from both the top and bottom of the weld as well as increased nugget size decreasing the Zn concentration to where brittle Fe-Zn phases do not form. Full penetration welds are recommended to reduce residual stress as well as allow sufficient outgassing of vaporized Zn in the weld pool.
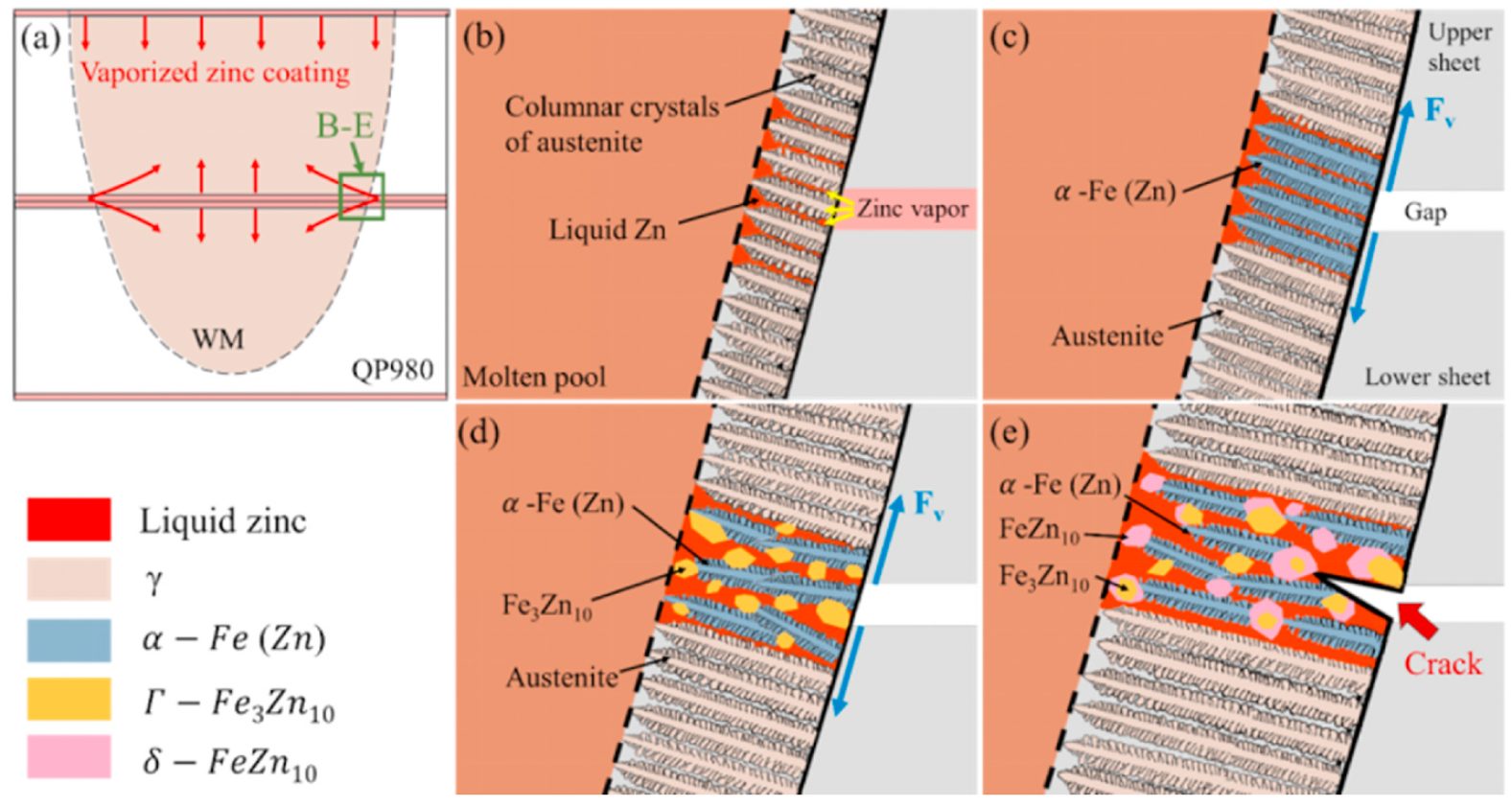
Figure 2: LME illustration.Z-11
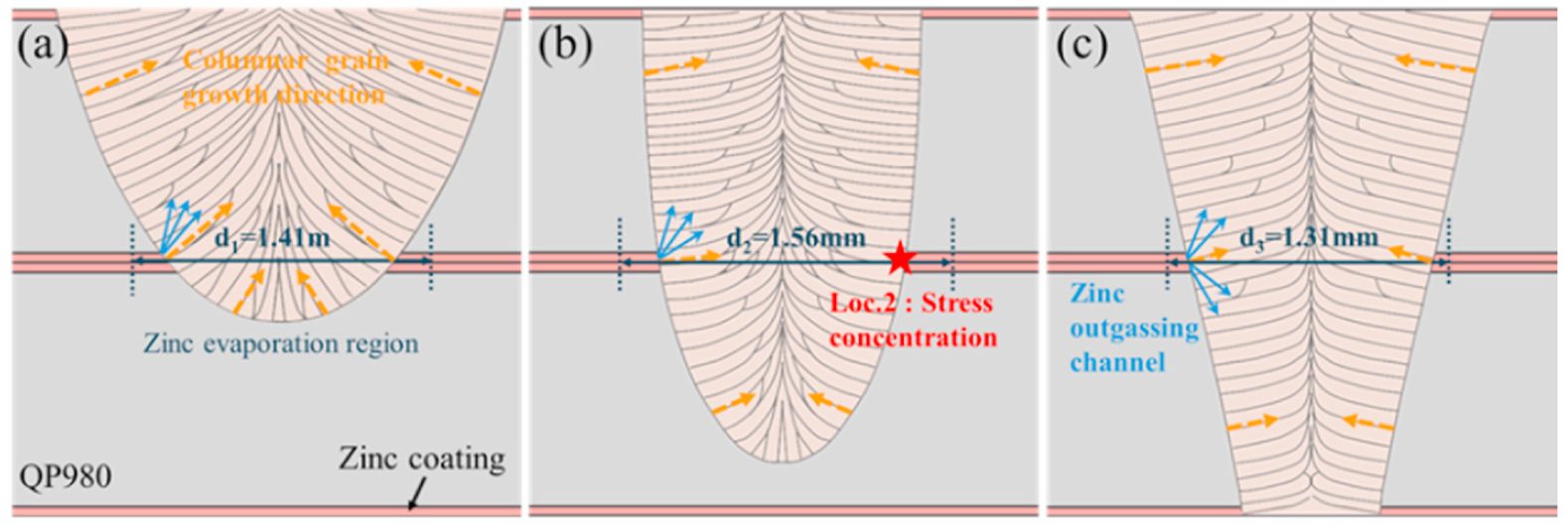
Figure 3: Zn diffusion.Z-11
Summary
LME cracks initiated at the weld notch root on the faying surface and propagated towards the center of the weld along the direction of columnar growth. Brittle intermetallic help induce cracking in the weld metal in addition to restraint from the weld joint. Full penetration welds were found to reduce Zn content from the weld in addition to reducing restraint as compared to partial penetration welds. The full penetration weld allowed for zinc to be outgassed from the weld on both sides reducing the Zn content in the weld to an acceptable level. Full penetration welds also reduced the residual stresses that formed during partial penetration welds due to the restraint on the root side of the weld.