AHSS, Blog, main-blog, News
Net Zero Emissions by 2050 – it’s a goal for future mobility that can seem distant and daunting. But over the past five years, WorldAutoSteel’s global automotive steel suppliers have conducted extensive research that illuminates a path forward. The Steel E-Motive concept – borne of this research – can be a catalyst for reaching the Net Zero goal.
Urbanization and changing attitudes towards vehicle ownership point to new transport opportunities in megacities worldwide. Mobility as a Service (MaaS) – characterized by autonomous, ride-sharing-friendly EVs – can be the comfortable, economical, and sustainable transportation solution of choice thanks to the benefits that modern steels offer, which will foster the higher vehicle occupancy that is critical to Net Zero ambitions.
Here, we break down the many benefits of the Steel E-Motive vehicle.
The Key Steel E-Motive Vehicle Features for Future Mobility
The Steel E-Motive Vehicle features seven key Advanced High-Strength Steel structural innovations to create a safe, economical vehicle.
- A B-Pillarless open-body structure offers excellent comfort, accessibility and easy ingress/egress.
- The Short Front Crash Zone design meets all global high-speed frontal crash requirements.
- The AHSS Extended Front Passenger Protection Zone provides excellent cabin intrusion protection for occupants.
- The Small Offset Crash Glance Beam minimizes the energy pulse into the occupant cabin, reducing the potential for passenger injuries.
- Hex beam energy absorbers provide superior battery protection for both side pole and deformable barrier crashes.
- The Scissor Door with Virtual B-Pillars offers excellent passenger visibility while saving mass and costs.
- The Coverless Battery Carrier Frame concept rewards 37% mass savings over benchmarks and 27% cost reduction; it also affords enhanced battery protection from road debris and other floor impacts.
The Steel E-Motive vehicle is created to meet Level 5 autonomy, meaning it is void of driver interfaces and does not require any human attention. With all of these features and more, the SEM architecture affords a spacious, safe, and comfortable cabin for occupants.
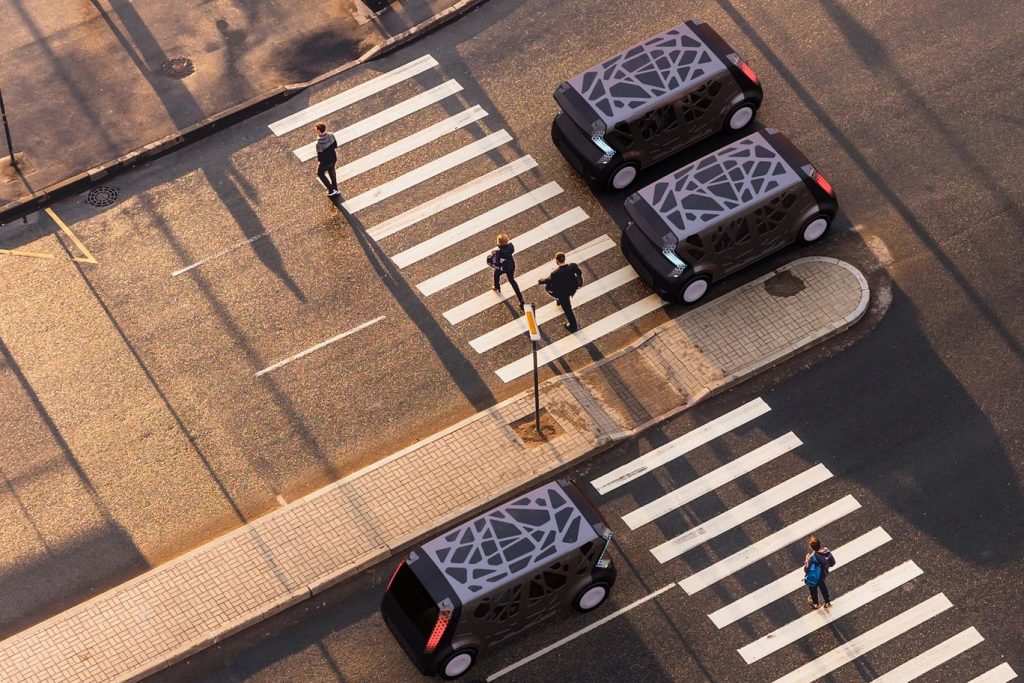
Steel E-Motive concepts are designed to help pave the way to a Net Zero future.
Exceeds Crash Guidelines
The Steel E-Motive vehicle is one of the world’s first autonomous vehicle concepts to validate and report excellent performance measured against the most stringent global crash requirements, which aligns with an IIHS “Good” rating. Modern Advanced High-Strength Steel product and fabrication process innovations enable the vehicle design to exceed these stringent crashworthiness standards while minimizing overall mass and production emissions.
Created to Be Affordable
Considering both production and life cycle costs, Steel E-Motive concepts have low maintenance requirements and are designed to be manufacturable using the world’s global manufacturing infrastructure at costs that support profitable margins, both for the vehicle manufacturer and the mobility service providers. Steel E-Motive is a fully engineered vehicle program that start-up companies can use to significantly reduce their cost and time to market.
Designed with Sustainability in Mind
The viability of any MaaS disrupter is contingent on cost competitiveness versus existing solutions, such as private ownership or taxis.
Moreover, our designs minimize steel thicknesses for lower mass while maximizing material utilization for lower steel production and emissions. Overall, the vehicle design offers the potential for ~86% CO2 emissions reduction when all factors contributing to sustainability are optimized. Autonomy further reduces operating emissions due to drive cycle smoothing.
To achieve our Net Zero future, high-occupancy vehicle usage is crucial and must be appealing for riders and profitable for providers.
Steel E-Motive concepts play a vital role in enabling Future Mobility Solutions THAT ONLY STEEL CAN MAKE REAL. Learn more about the program: https://steelemotive.world/
Blog, main-blog
The Beginnings of PHS Use
Press hardening, as we know it today, was developed in Luleå, Sweden, by Norrbottens Järnverks AB (abbreviated as NJA, translated as Norrbotten Iron Works). The first patent application was completed in 1973 and awarded in 1977.N-23 The technology was first commercialized in agriculture components, where the high strength of Press Hardened Steels (PHS) was favored for wear resistance.B-45
In 1984, automotive applications of PHS started with the Saab 9000 side impact door beams, as seen in Figure 1. A total of 4 parts were used in this car.A-66 The uncoated blanks were almost half the thickness of a cold stamped beam.T-26
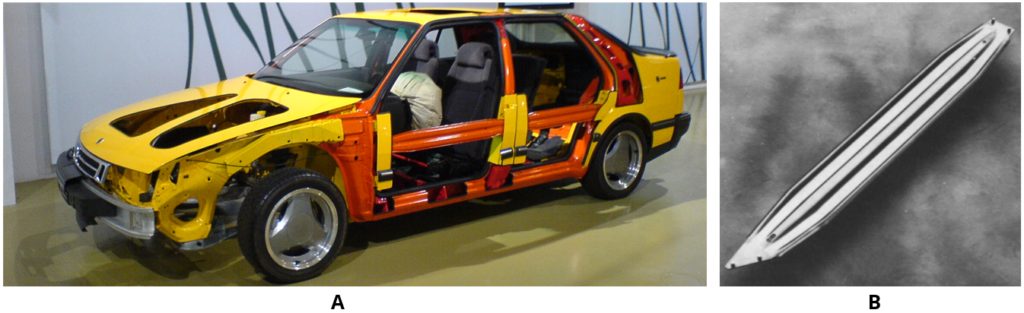
Figure 1: Door beams of the Saab 9000 (1984-1998): (a) A see-through car in Saab MuseumS-82, (b) the hot stamped part.L-42
The majority of the PHS parts were door beams through the mid-1990s, with approximately 6 million beams produced in 1996. By this time, the demand for bumper beams was also increasing.F-31 By the end of 1996, the European New Car Assessment Program (EuroNCAP) was formed, which increased the pressure on the OEMs for improved crashworthiness.T-26 In 1998, both the new Volvo S80L-44 and Ford Focus5 were equipped with Press Hardened bumper beams.
The year 1998 saw the development of one of the most important breakthroughs in Press Hardening technology. French steel maker Usinor developed an aluminum-silicon (AlSi) pre-coated steel, commercialized as Usibor 1500 (indicating the typical tensile strength, 1500 MPa.C-24, L-39 In 2000, BMW rolled out its new 3 series convertible. In this vehicle, the A-pillar is made from 3 mm thick uncoated, PHS sheet. This was BMW’s first PHS application, and one of the first PHS A-pillar reinforcement.S-83, S-84 Accra started delivering roll formed PHS components for the Volvo V70, initially an optional 3rd row seating support. Approximately 10,000 parts/year were supplied.G-28
AlSi coated steel was first hot stamped at a French Tier 1 supplier, Sofedit.V-15 This grade was first used in the front bumper beam of the 2nd Generation Renault Laguna (2000-2007). Laguna 2 was the first car to receive a 5-star safety rating from Euro NCAP.V-10 AlSi coated blanks were also used in PSA Group’s Citroën C5 (1st Gen: 2001-2007) in the front bumper beam, and the A-pillars. These three parts weighed a total of 4.5 kg, approximately 1% of the total BIW weight, Figure 2a. About one month later, PSA Group started production of the compact hatchback Peugeot 307, which had five hot stamped components (A- and B-pillars and rear bumper beam). Unlike the Citroën C5, these parts were uncoated. The total weight was 12 kg, corresponding to 3.4% of the BIW weight.R-17, P-27
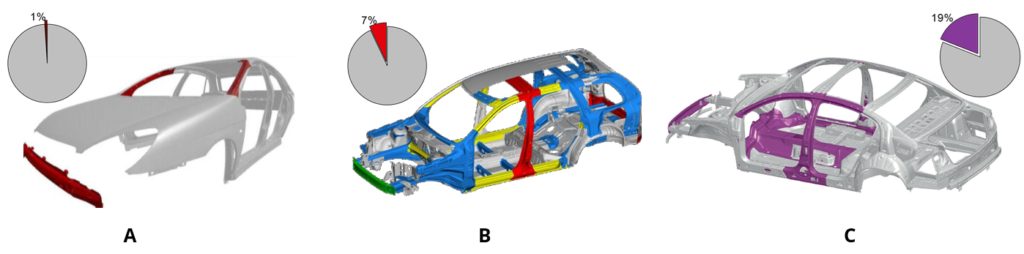
Figure 2: Increase in press hardened component usage: (a) 2001 Citroën C5P-27, (b) 2002 Volvo XC90L-29 and (c) 2005 VW Passat.H-50
Volvo started producing the XC90 SUV in 2002. The body-in-white with doors and closures weighed 531 kg.B-44 A total of 10 parts, weighing 37 kg are either roll formed or direct stamped PHS. This accounts for approximately 7% of the BIW weight.L-43 During its time, this was the highest use of PHS in car bodies. In Figure 2b, the Press Hardened components other than the 2nd row seat frame, which is a load bearing body part, are shown.
Accelerated Use and Globalization
The use of press hardened parts increased rapidly after the introduction of the VW Passat in 2005. This car had approximately 19% of its BIW (by weight) made from press hardened steels, Figure 2c. Some parts in this car saw the first use of varnish coated blanks in a two-step hybrid process. Three parts were produced using either an indirect or hybrid process, including the transmission tunnel.H-50
Following are a few highlights of PHS use in vehicle applications during this time period :
- In 2006, the Dodge CaliberK-37 and BMW X5P-28 were among the first cars to have tailor-rolled and Press Hardened components in their bodies (Figure 3).
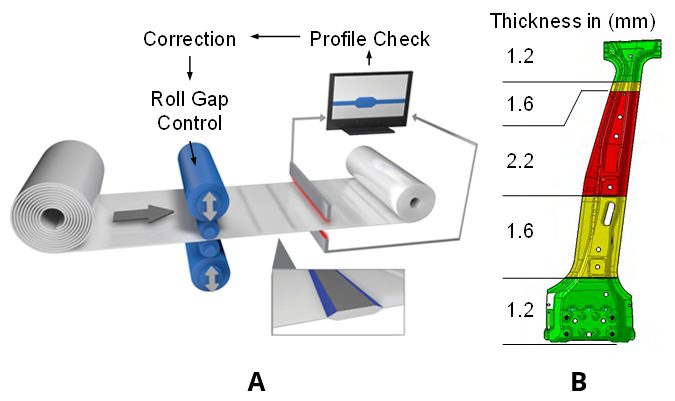
Figure 3: (a) Tailor Rolling ProcessZ-5, (b) B-pillar of BMW X5 (2nd Gen: 2006-2013).P-28
- BMW 7 Series (5th Gen: 2008-2015) became the first car to have Zn-coated Press Hardened components in its body-in-white. The car also contained uncoated parts, as shown in Figure 4 (next page). The total PHS usage in this car was approximately 16%.P-20
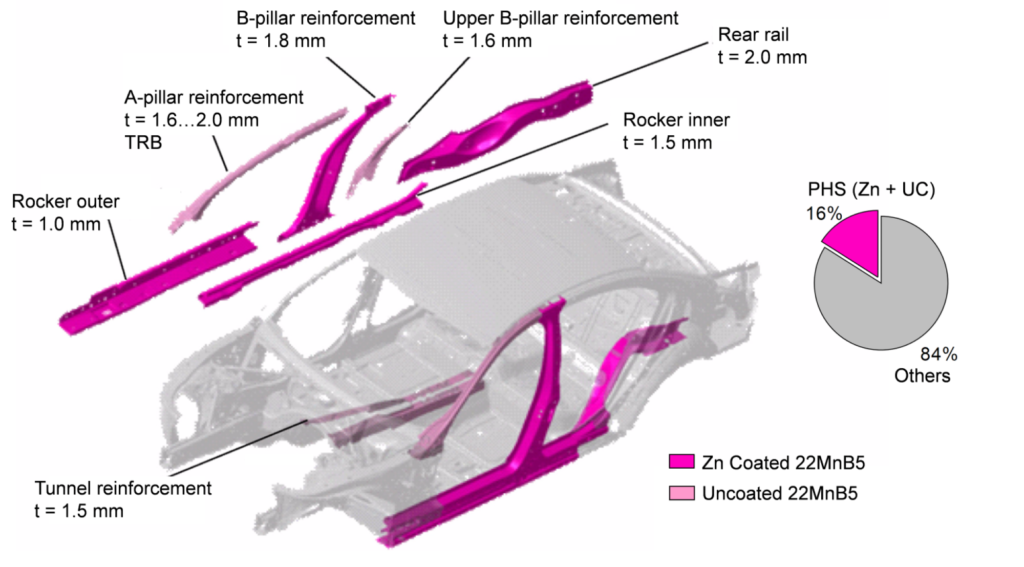
Figure 4: PHS usage in BMW 7 Series (5th Gen: 2008-2015) (re-created using P-20).
- Press hardening also allowed car makers to create unconventional cars. In 2011, Hyundai rolled out the 1st generation Veloster, a 3-door coupé (also known as 2+1, with one door on the driver side and 2 doors on the passenger side), and as such containing axisymmetric front doors. Thus, the car could not have a full B-ring, as illustrated in Figure 5a.B-14, R-19 Another unconventional design was the Ford B-Max subcompact MPV sold in Europe between 2012 and 2017. The car had conventional swing doors in the front and two sliding rear doors. A PHS B-pillar was integrated in the doors, providing ease of ingress. Its PHS components (integrated B-pillar in front and rear doors, door beams and cantrail) are shown with blue color in Figure 5b.B-14, L-45
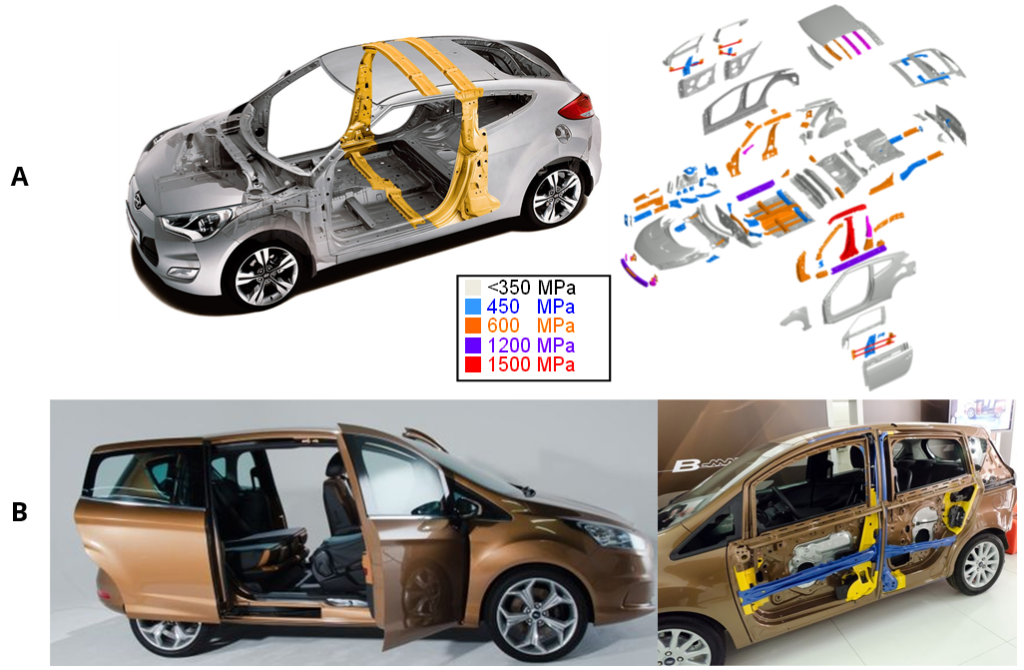
Figure 5: Unconventional car designs with PHS: (a) Hyundai Veloster, asymmetric 2+1 doors coupé (re-created after Citation R-19), and (b) Ford B-Max, sub-compact MPV with integrated B-pillars in the doors.L-45
In 2013, the Acura MDX (3rd Gen: 2013-2020) became the first car to have a Hot Stamped door ring. The part was a tailor welded blank comprised of two sub-blanks, as shown in Figure 6a. The design saved about 6.2 kg weight per car and had high material utilization ratio thanks to sub-blank nesting optimization.A-67, M-46 One of the most recent PHS applications was in 2017 Chrysler Pacifica with 5 sub-blanks, as shown in Figure 6b. This car also has a PQS550 sub-blank at the lower B-pillar region.D-28
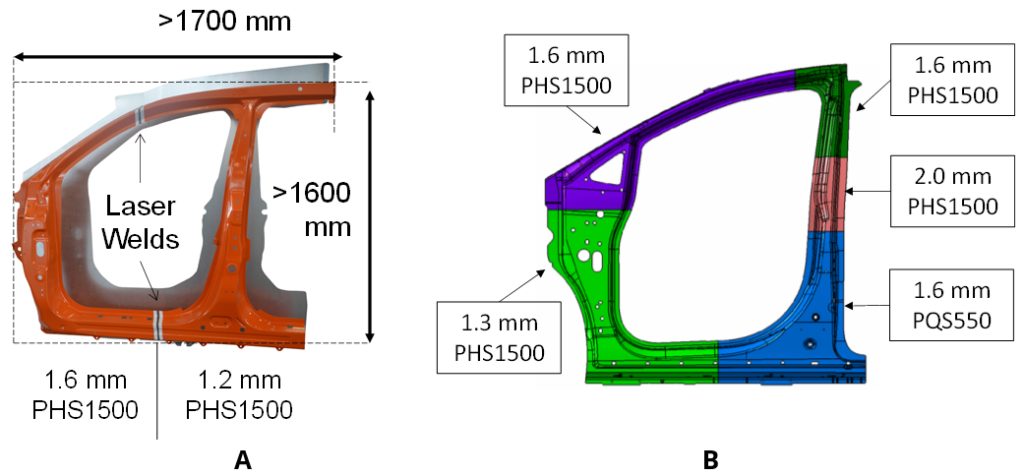
Figure 6: Hot stamped door rings: (a) First application in 2013 Acura MDX had 2 sub-blanks, (b) a more recent application in 2017 Chrysler Pacifica has five sub-blanks with PQS550 at the lower B-pillar (re-created after Citations B-14, A-67, D-28).
- Tubular hardened steels have been long used in car bodies, with minimal forming. Since 2013, a special 3-D hot bending and quenching (3DQ) process has been employed. One of the earliest uses of this technology was Mazda Premacy (known as Mazda 5 in some markets). The same process was also used in making the A-pillars of the Acura NSX (Honda NSX in some markets, 2016-present), as seen in Figure 7a.H-29 Since 2018, tubular parts formed with internal pressure, called form blow hardened parts, are used in the Ford Focus (4th Generation) (Figure 7b) and Jeep Wrangler (4th Generation).B-16, B-17
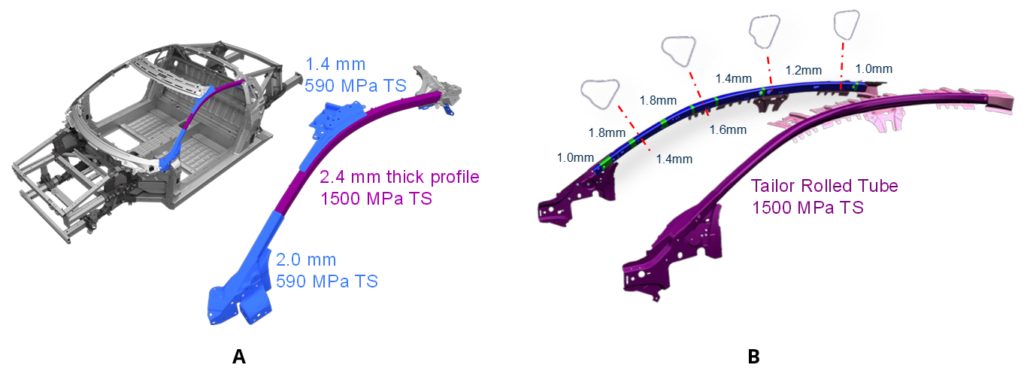
Figure 7: Tubular hardened steel usage in A-pillars of: (a) 2015 Acura NSXH-29, (b) 2018 Ford Focus.B-16
PHS Use in xEVs: Hybrid Electric, Battery Electric,
Plug-in Hybrid Electric & Fuel Cell Electric Vehicles
The first commercially available Hybrid Electric Vehicle (HEV) was the Toyota Prius (1st Gen: 1997-2003). The second-generation Prius (2003-2009) had very few Press Hardened components, as shown with red color in Figure 8a. This was the first time Toyota used hot stamped components.M-47 The third generation Prius (2009-2015) had approximately 3% of its BIW Press Hardened. In the 4th generation Prius released in 2015, the share of >980 MPa steels has risen to 19%.U-10 Figure 8b shows the Press Hardened parts in this latest Prius.K-38
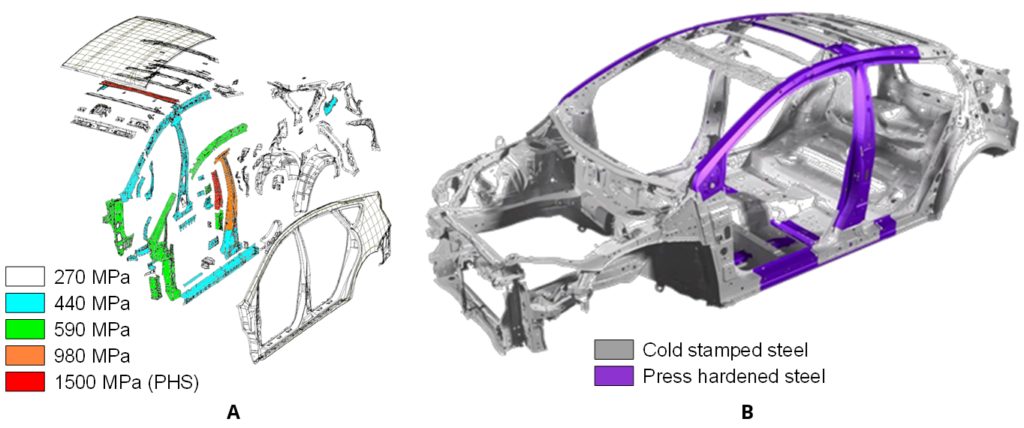
Figure 8: PHS usage in Toyota Prius: (a) 2nd generation (2003-2009) and (b) 4th generation (2015-present) (re-created after Citations M-47 and K-38)
The 2012 Tesla Model S and Model X launched using aluminium bodies, with PHS reinforcements in the pillars and the bumpers. Model S is known to have a roll-formed PHS bumper beam. High volume Model 3 and Model Y have a significant amount of press hardened components in their bodies.T-35
In 2011, General Motors started production of its first Plug-in Hybrid Electric Vehicle (PHEV), the Chevrolet Volt (known as Opel Ampera in EU and Vauxhall Ampera in the UK). This car had six Hot Stamped components, including A and B pillars, accounting for slightly over 5% of the BIW mass.P-29
The smaller BEV Chevrolet Bolt, launched in 2017, had aluminum closures, but a steel-intensive BIW that is 80% steel, 44% of which is Advanced High-Strength Steels including 11.8% PHS. Figure 9.A-69
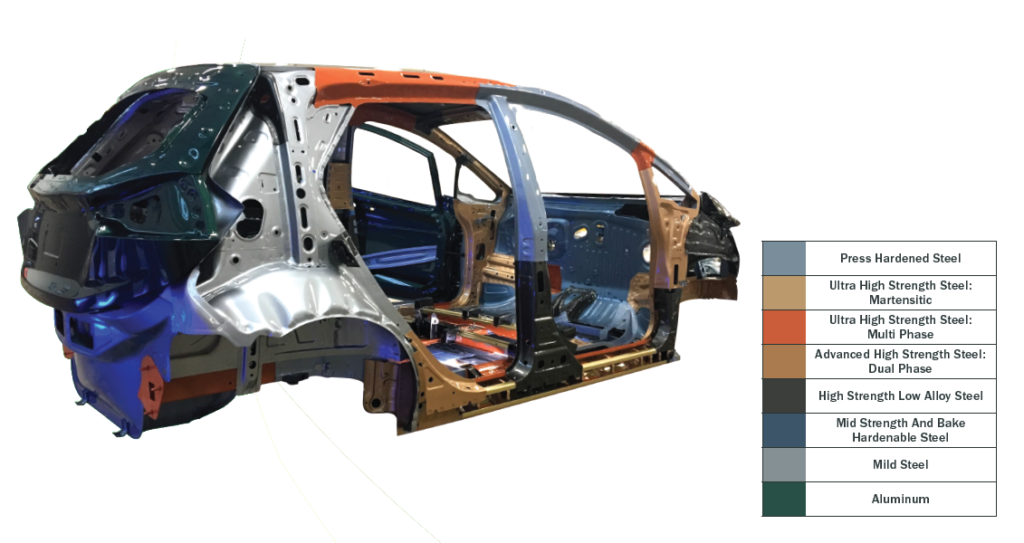
Figure 9: Chevrolet Bolt Body Structure and Steel Content.A-69
In December 2020, Hyundai announced their new electric platform, E-GMP. The platform will utilize Press Hardened steel components to secure the batteries.H-52
Automakers have turned to PHS to manage the extra load of Fuel Cell powertrains as well. The first-generation Toyota Mirai had only Press Hardened B-pillars, cantrails and lateral floor members.T-38 The second generation has a number of parts with PHS in its under body as well.T-39
In 2018, Hyundai Nexo became the first fuel-cell car to be tested by EuroNCAP, achieving a 5-Star rating. The car has PHS A- and B-pillars, rocker reinforcements, and several under body components, as seen in Figure 10.H-53
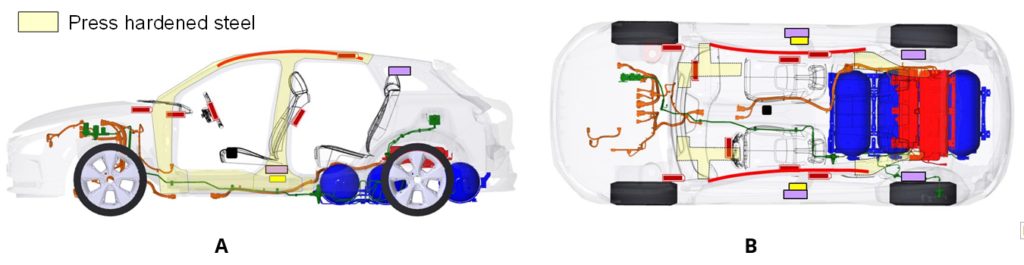
Figure 10: Press hardened steel usage in Hyundai Nexo Fuel Cell vehicle: (a) side view and (b) top view (re-created after Citation H-53).
Did you enjoy this story? You can find a much more detailed article here in the Guidelines with many more vehicle examples and history data.
Blog, main-blog
Several recent studies are forecasting that; “Within the next 10 to 15 years, urban transportation will be dominated by Electric and Automated vehicles”.B-50 Meaning most of us will be driving Battery Electric Vehicles (BEVs) in the not-distant future. In 2011, just eight years ago, there were only three BEVs on the market with 70 to 80 miles range on a single charge. These were the first generation BEVs. Since then, the number of EVs on the market has increased, with significant improvements in range (now approaching 300 miles). BEV 2020 vehicles cover all current segments, from small cars to SUV’s and trucks (Figure 1). These vehicles will be available from most OEMs as well as several new start-up companies. The construction material for body structures of these vehicles is predominantly steel, while some of the premium vehicles ($60,000 to $100,000) are aluminium. And the prevailing OEM message seems to be “anything TESLA can do, we can do better”.
So how will this change the vehicle body structure design, choice of construction material, its implications for manufacturing and assembly, and ultimately, the impact on automotive steel?
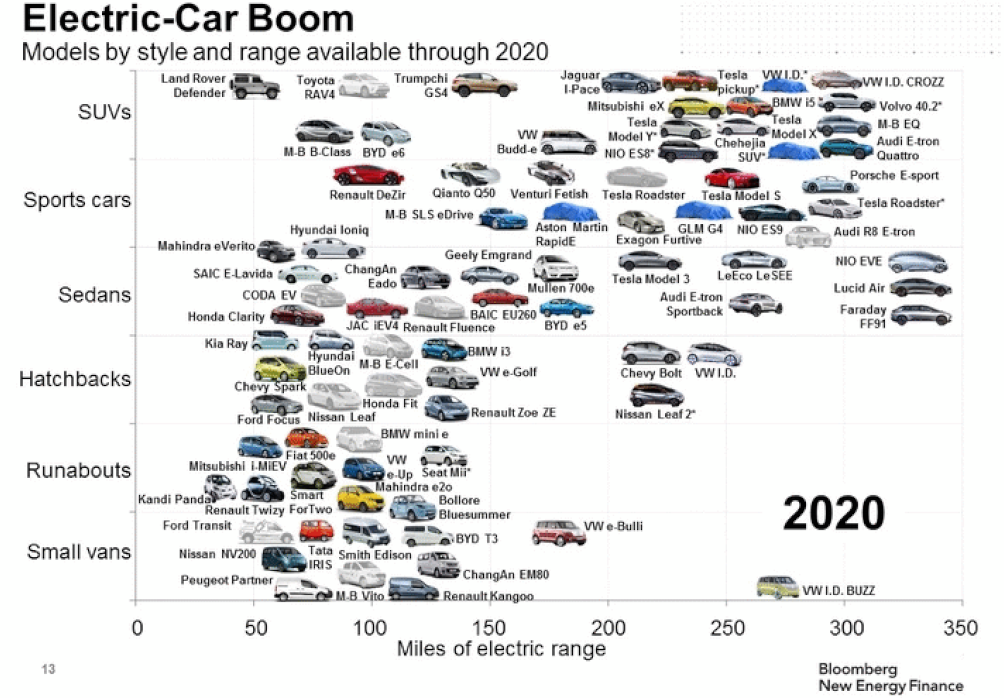
Figure 1: Electric Vehicle Boom – Models by Style and Range Available Through 2020.B-50 CHART SUMMARY: a) Covers all current segments, b) Structures predominantly Steel, c)Some premium vehicles highlight Aluminium, d)Products from most OEMs as well as several new start-up companies.
The driver for this electrification boom is increasing affordability. The upfront cost of BEVs will become competitive on an unsubsidized basis starting in 2024.F-38 By 2030 in the U.S., almost all light duty vehicle segments will reach cost parity as battery prices continue to fall.B-73 Forecasters, such as McKinsey, Morgan Stanley and Bloomberg, predict that about half of all new vehicle production will be electric somewhere between 2035 and 2040. However, Tesla’s CEO Elon Musk’s prediction is much more aggressive. He expects more than half of new vehicles in the U.S. will be electric within the next 10 years, roughly 10 to 15 years ahead of most other predictions.
The Main Drivers of BEV Cost Reduction
- Lithium-ion battery prices have fallen 75% since 2013, hitting $176/kWh in 2018 (Figure 2). Industry-wide prices fell due to the adoption of new cell designs and the availability of higher energy-density cathodes. Prices are expected to drop further in coming years to below $100 per kWh. Besides the reduction in cost, packaging efficiency and the cell energy density also is improving.
- Package space required by other BEV powertrain systems also is being optimized, e.g., motor, transmission, differential and power electronics. This is yielding significant weight and cost reductions, which are then directly reinvested into lower-cost structural materials, such as Advanced High-Strength Steels (AHSS) versus higher cost Aluminium, to keep the overall price of the vehicle low.
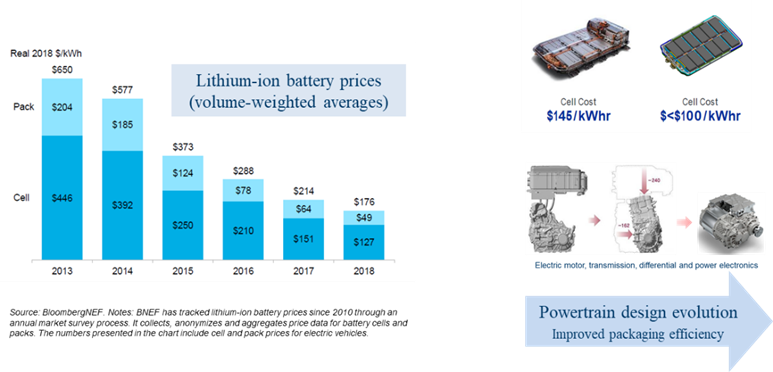
Figure 2: BEV Price Parity with Gas-powered Cars by 2024 – Main Drivers.B-74
BEV to ICE Vehicle Structural Differences and Advantages for Steel
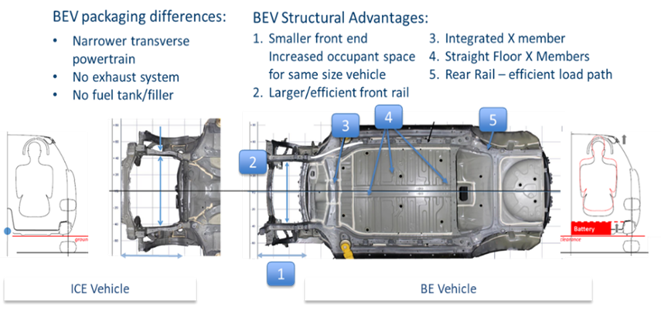
Figure 3: BEV to ICE Vehicle Structural Differences.M-64
BEV packaging differences compared with ICE Vehicles are shown in Figure 3, and include:
- Narrower and compact transverse electric powertrains, leading to shorter front end, with increased occupant space for same size vehicle and larger/efficient front crash rails.
- Lack of an exhaust system eliminates the need for the tunnel, allowing straighter/ efficient cross-members.
- No fuel tank/filler leads to more efficient rear rail load path.
- High voltage electric powertrain and large (300 litres, 500 kg) under-floor battery pack crash protection requirements result in higher safety requirements for BEV front and side structures.
- Safety. The BEV body structure load path requirements are ideal for AHSS application. The floor cross members, without the presence of the tunnel, are straight and can use very high-strength martensitic roll formed sections. Cross members can be stamped from 3rd Generation Steels offering Giga-Pascal strength and over 20% elongation. For frontal crash load management and to minimize passenger/battery compartment intrusions for increased safety, 3rd Generation steels offer the most mass/cost efficient solution. The very high strengths offered by AHSS and UHSS for the safety-critical structural members such as the rocker, rails, cross members and pillars, greatly enhance the required protection of the BEV powertrain and high energy/voltage battery systems. The battery enclosure construction greatly benefits from AHSS usage, providing protection from road-debris impacts from below the vehicle, along with fire protection into the passenger compartment. Advanced steels also enable reduced section sizes for the occupant compartment, required for improved panoramic visibility, without compromising occupant safety and comfort.
- Cost. For widespread adoption of BEVs to occur, the overall cost of the vehicle must be affordable, and its range must be above the ‘range anxiety limit’ of most drivers. Various surveys indicate this range to vary greatly from 75 miles to over 400 miles. Using steel for the vehicle structure leads to the lowest cost BEV, just as with ICE-based vehicles. The vehicle range can be increased through lightweighting and/or by increasing the size of the battery; a cost comparison of these two options is shown in Figure 4. With battery cost reduction approaching $100 per kWh, lightweighting is cost effective at approximately US$2.00 per kg saved. Lightweighting is still very important and the latest steel grades, in particular 3rd Generation steels, offer the most cost-effective lightweighting option. In comparison, if we consider lightweighting with aluminium, the cost is typically in the order of US$6.00 per kg saved. This could be cost effective if the battery cost is over $250 per kWh, which was the case a decade ago. We can see the evidence of this in OEM decisions at that time. For example, the 2011 Nissan Leaf BEV closures were aluminium; but the latest 2019 Nissan leaf BEV closures are steel.
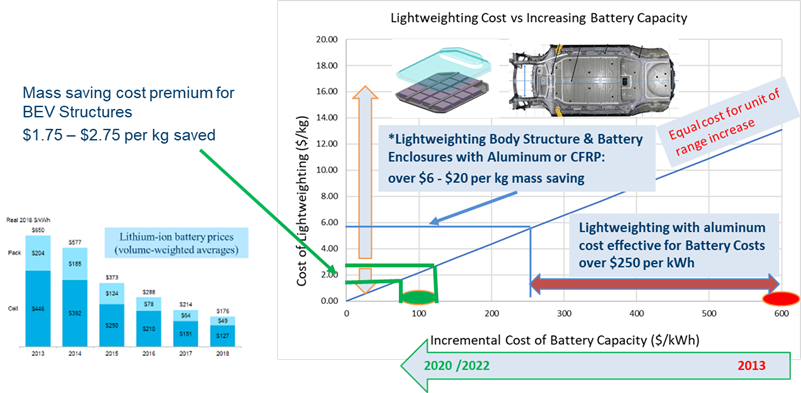
Figure 4: BEV Range Increase – Lightweighting Cost versus Battery Cost 2020 – 2022.M-64
Battery Electric Vehicles – Boom or Bust for AHSS?
For the increased safety required for BEVs to protect the high voltage systems, the structural load paths are ideally suited for the Giga Pascal level strengths offered by AHSS and UHSS. The Battery Enclosure structure offer an additional 85 kg per vehicle opportunity, an increase of approximately 10% sheet metal over ICE vehicles. Also, using advanced steels the BEV structure can take full advantage of well-established body shop practices for manufacturing and assembly, such as stamping, roll forming and spot welding. With future increased focus on BEV affordability, safety and sustainability, steel offers the best solutions and flexibility to address these key challenges.
Blog, main-blog
Here at WorldAutoSteel, we have been studying the changes in the automotive industry for several years, focusing particularly on ride sharing, autonomous, electric vehicles and steel’s role in that marketplace. George Coates, Technical Director for WorldAutoSteel and The Phoenix Group, has been leading that effort and today contributes an article on the disruption of future mobility to the industry and the great opportunities we see for steel in meeting the challenges providers will face. We hope you enjoy the read, and we welcome your thoughts and comments. What changes and impacts do you envision for vehicle manufacture? How do you feel about the world of autonomy?
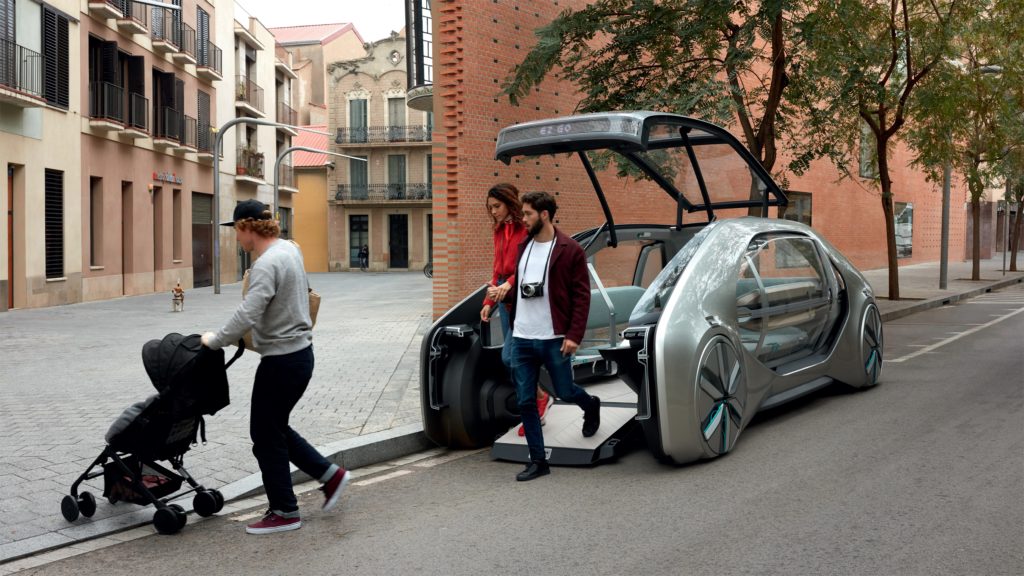
Renault’s Future Mobility concept, the E-Z Go
We’re approaching a critical milestone in automotive history when what we know as normal is about to change significantly. Future Mobility describes the revolution that’s already begun. We’re rethinking transportation from the movement of a vehicle to a more efficient concept for moving people and things. We’re about to discover the social advantages of connected, autonomous, shared and electric vehicles. And we’re completely changing the way we view transportation.
By 2030, electric vehicles (EVs) will be mainstream—not just within the premium segment, as they are today. EV’s will be popular and available across all vehicle variants and prolific in the commercial vehicle industry and in public transportation. Owners and fleet providers will experience the lower costs of electricity, lower maintenance costs, and the lower overall total cost of ownership (TCO). Fully autonomous or self-driving vehicles will introduce design freedom never experienced before, with the removal of the steering wheel, foot pedals and conventional dashboard. Communication and comfort will be re-imagined, with a vehicle that’s no longer designed around the driver but designed to serve the needs and comfort of the occupants, who are now users instead of owners.
With the rise of mobility services such as Uber, Didi, and a host of others, vehicle ownership is fast becoming an option. In a very short time, especially in urban areas such as China’s mega-cities, it is becoming cost-efficient to subscribe to a monthly ride share service for all of your transportation needs.
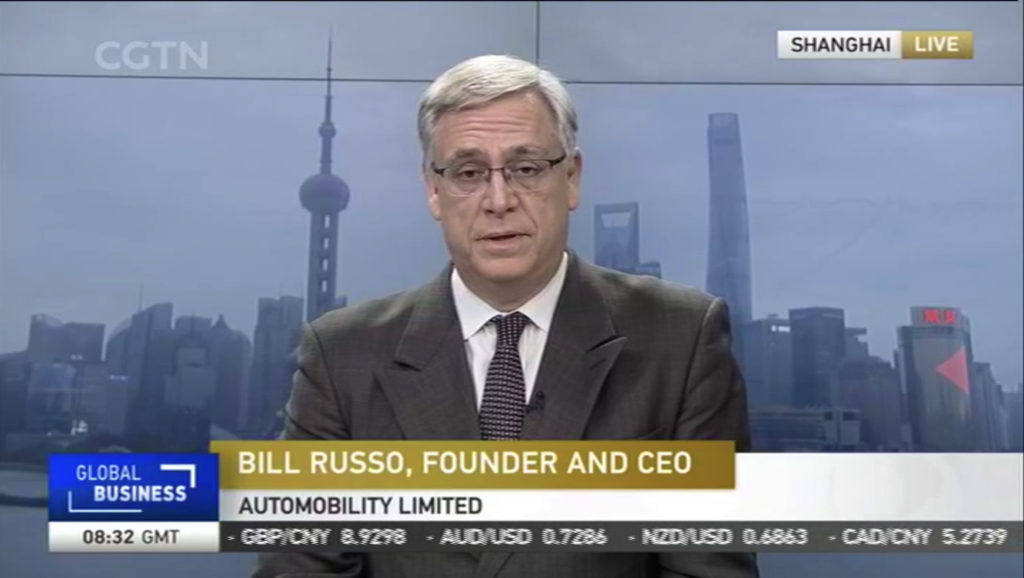
Bill Russo, CEO, Automobility LTD
Bill Russo, CEO of China-based Automobility, in a December 2018 article, Competing in the Digital Internet of Mobility, notes that the digital connectivity of these vehicles will open up profit opportunities well beyond the vehicle hardware. He says “An expanded understanding of mobility use cases and tailoring of the mobility hardware ‘form factor’ to the particular mobility need will be a way to create a value proposition that is rooted in the unique riding experience. In the user-centric world where users are passengers, the focus shifts from traditional driver-centric design to a user-centric productivity space. Instead of traveling in the cockpit, we will move in business class or economy class, depending on our preferences and budget.” Cities will be re-imagined in new social opportunities associated with autonomy, as these vehicles will serve the under-served, and infrastructures will shift in purpose to move people, as opposed to moving vehicles.
Where does steel fit?
The steel industry plans to be right in the middle of this revolutionary change. Fleet owners who provide ride hailing and ride sharing services need to manage the total cost of ownership, while maximizing the user experience for added revenue. To be profitable, they’ll want durable, lasting structures that are affordable to own, provide the user motion as well as emotional comfort, while being efficient to operate, and environmentally friendly – and steel is the only material that meets all these requirements.
On Camera Now: George Coates, Technical Director, WorldAutoSteel and Phoenix Group from worldautosteel on Vimeo.
As always, steel is needed for the crash safety structures, and now add battery protection. Our market intelligence shows that due to the high cost to municipalities and regional governments, autonomous-only vehicles will be limited to dedicated areas for a long time to come. Meanwhile, vehicle-to-vehicle and vehicle-to-infrastructure connectivity will result in dramatic improvements in accident avoidance and reduced fatalities.
Because it will take many years before all vehicles on the road have these technologies in play, the need for passive safety will remain for the foreseeable future. Developing a structural design for the passenger compartment becomes challenging, since there’s a now a need to strike a balance between occupant safety and the occupant freedom. This is enabled by removing the driver and controls from the interior. Steel will be needed to provide the unique properties of both crash energy absorption and deflection, while also managing the loads associated with passengers in multiple and diverse seating configurations. Steel has the ability to provide needed strength while keeping the material thin, which lends more room in the passenger cabin for new seating arrangements and more seats. And battery housings made from steel will provide structural integrity for crash management, while also preventing battery pack damage and leakage.
Lightweighting will continue to be important in an effort to balance smaller battery sizes with maximum range. The steel industry has been and will continue to develop products, such as the ever-growing family of Advanced High-Strength Steels (AHSS), to meet both the mass reduction and the safety targets, affordably. With content innovation and the amazing flexibility of the Iron (Fe) element, researchers still have vast development possibilities for new steels that are stronger, more formable and cost effective.