RSW of Dissimilar Steel
This article is the summary of a paper entitled, “Weld Nugget Penetration of a Four-Sheet Resistance Spot Welding Advanced High-Strength Steels”, by K. Namola, et al.N-11
Experimental Weld Nugget Penetration
The study analyzes the effect of electrode size and composition on final weld nugget size and penetration. Nugget growth patterns were analyzed and weldability issues characterized. Figure 1 shows the arrangement of the four-layer stack-ups that were tested in this study. Truncated code electrodes used were a 6-mm Class 1, 6-mm Class 3, 6-mm Class 20, 8-mm Class 1, and 10-mm Class 1. Samples were welded in the as-received condition. JAC270 is a cold rolled Mild steel with a galvanneal coating having a minimum tensile strength of 270 MPa. JSC590 and JSC980 are bare cold rolled Dual Phase steels with a minimum tensile strength of 590 MPa and 980 MPa, respectively.
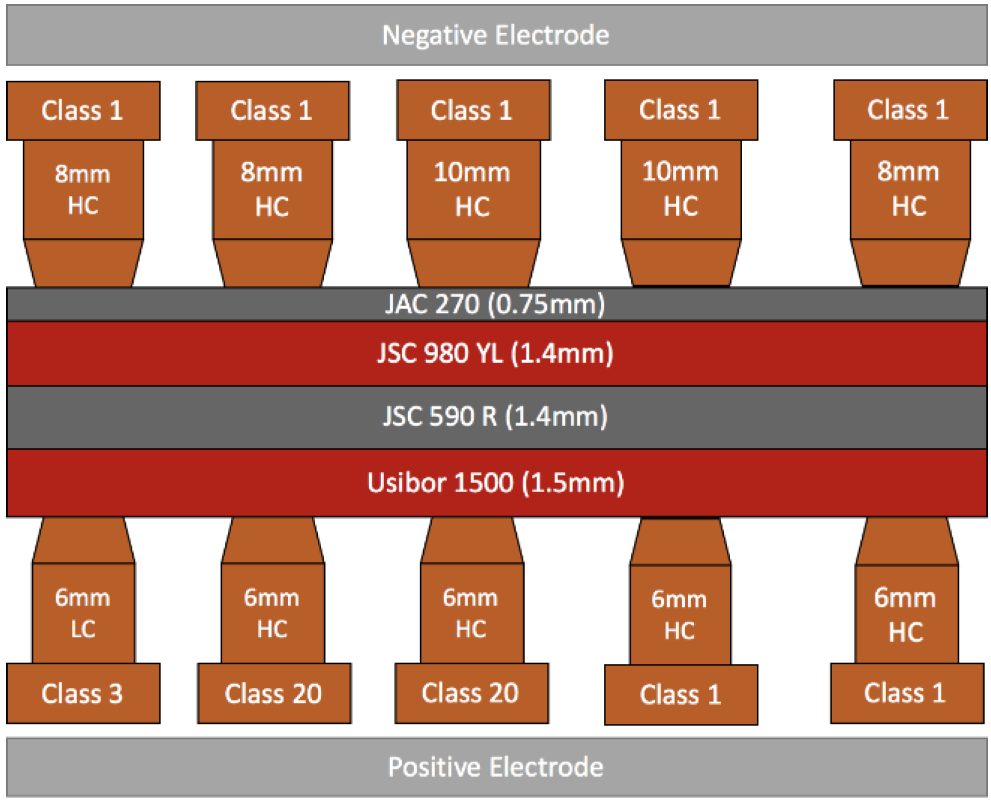
Figure 1: Resistance Welding Stack and Test Electrode Combinations.N-11
Best results from the iterative trials were obtained using an 8- and 6-mm Class 1 copper electrode with the weld schedule shown in Figure 2. This weld schedule was repeated using the electrode combinations listed in Table 1. Figure 3 shows cross sections of each weld listed in Table 1.
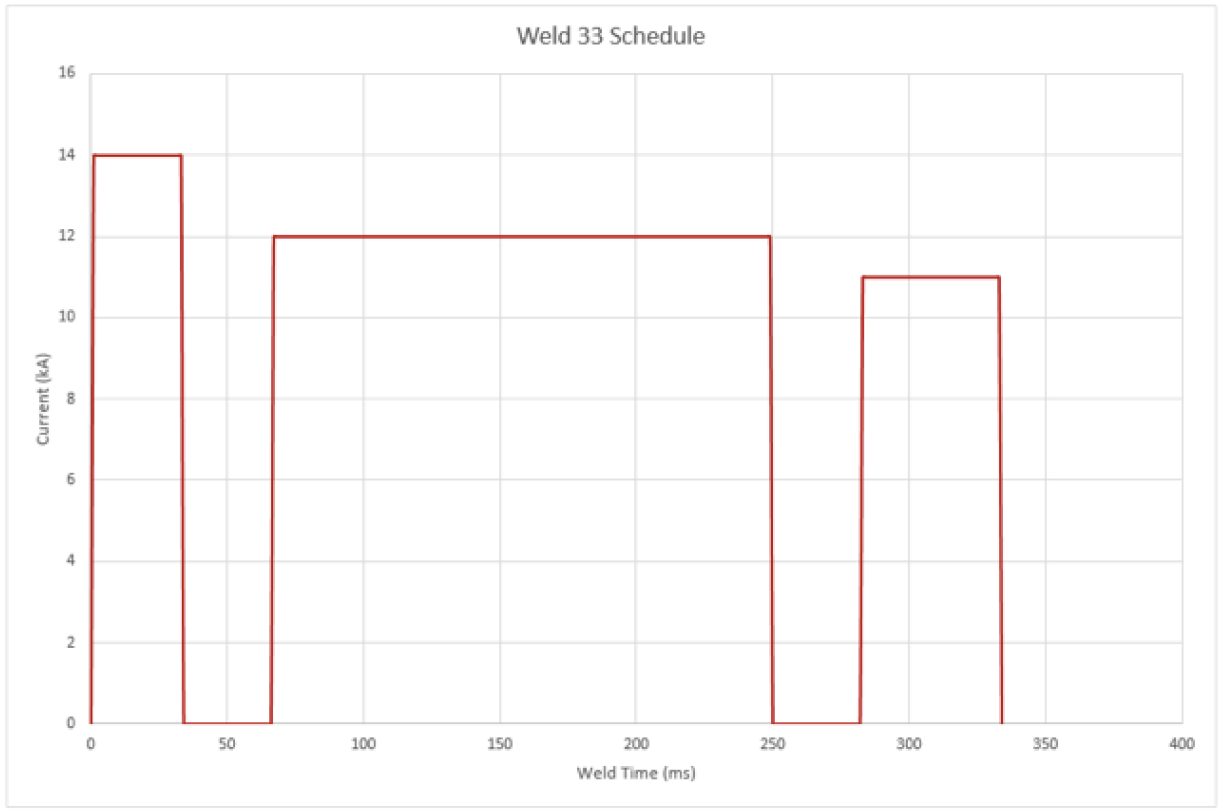
Figure 2: Down-Selected Weld Schedule from Trials.N-11
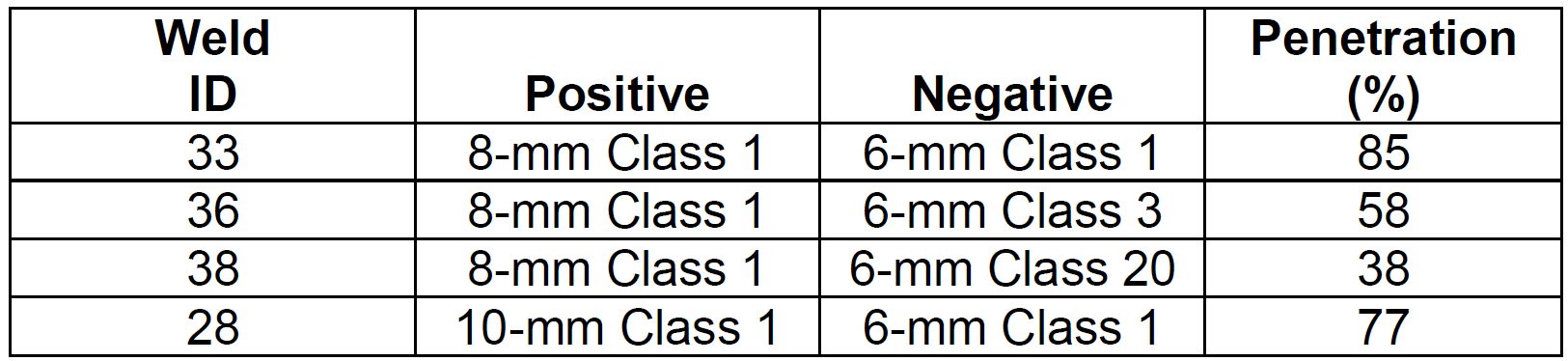
Table 1: Nugget Penetration Using the Down-Selected Weld Schedule from Trials and Different.N-11
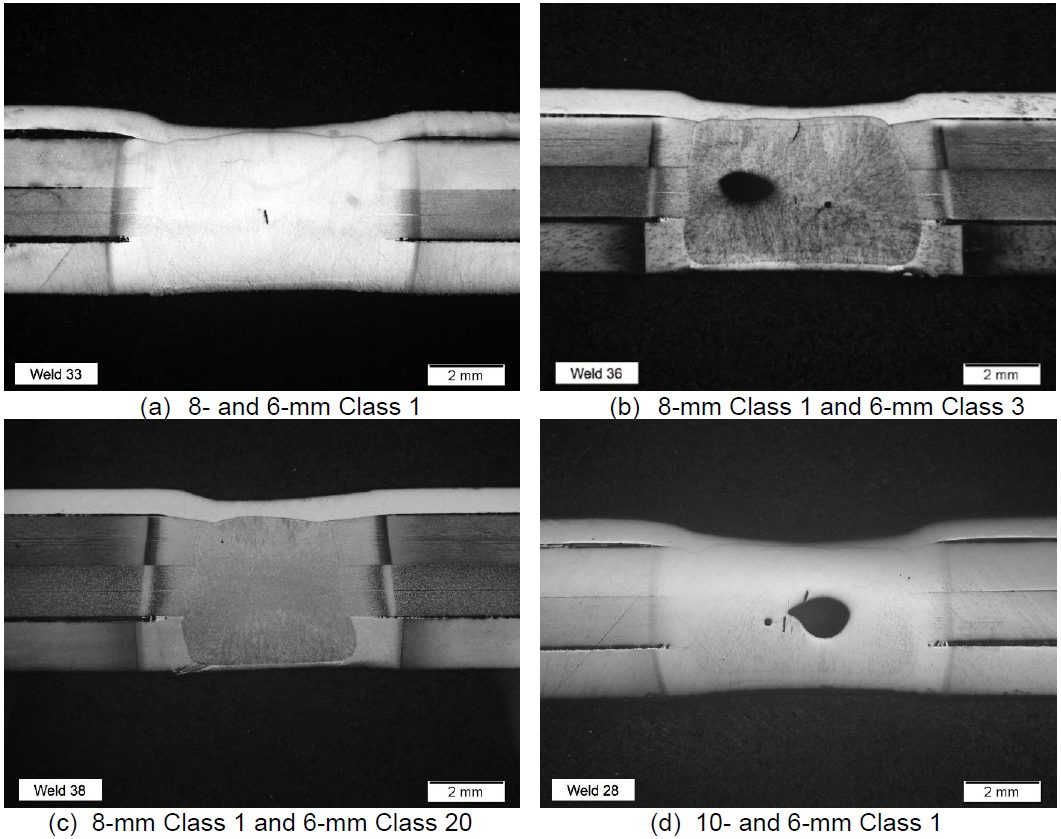
Figure 3: Welds Made Using the Down-Selected Schedule and Different Electrodes.N-11
Figure 4 shows cross sections of five welds made starting with new 8- and 6-mm Class 1 electrodes. As can be seen, expulsion gets progressively worse over time but penetration does not. Penetration values into the JAC 270 were determined by metallography and are shown in Table 2.
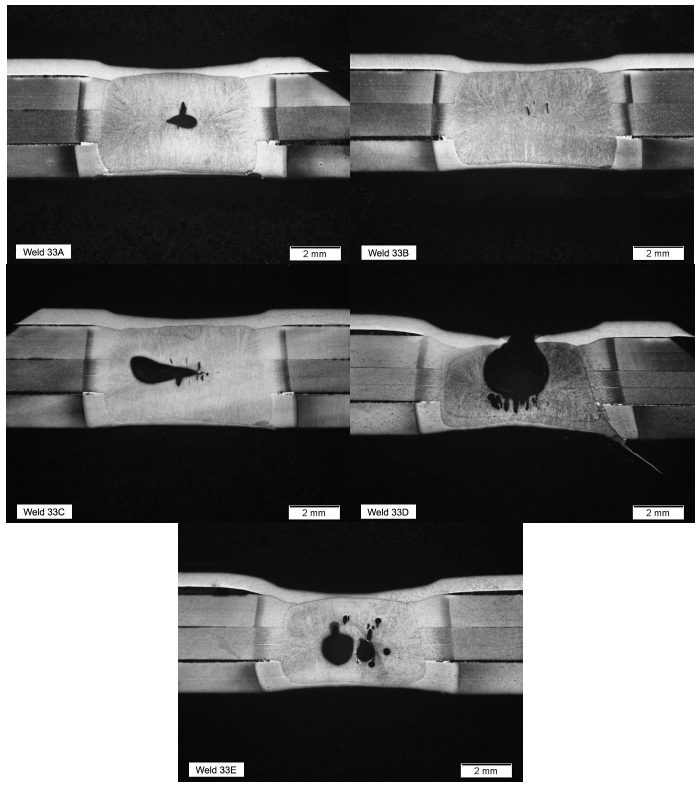
Figure 4: Welds from Repeatability Study Using the Down-Selected Weld Schedule and 8- and 6-mm Class 1 Electrodes.N-11
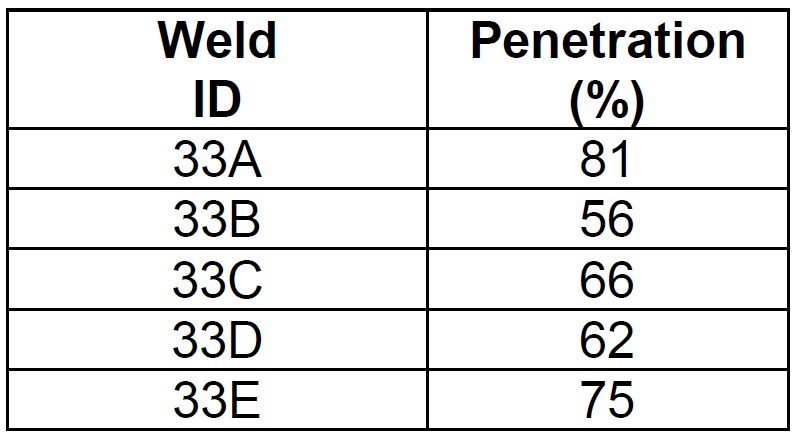
Table 2: Nugget Penetration into the JAC 270 During Repeatability Study.N-11
Table 3 lists the resistance measurements at the weld stack interfaces. Figure 5 shows the resistance graph of weld stack up.
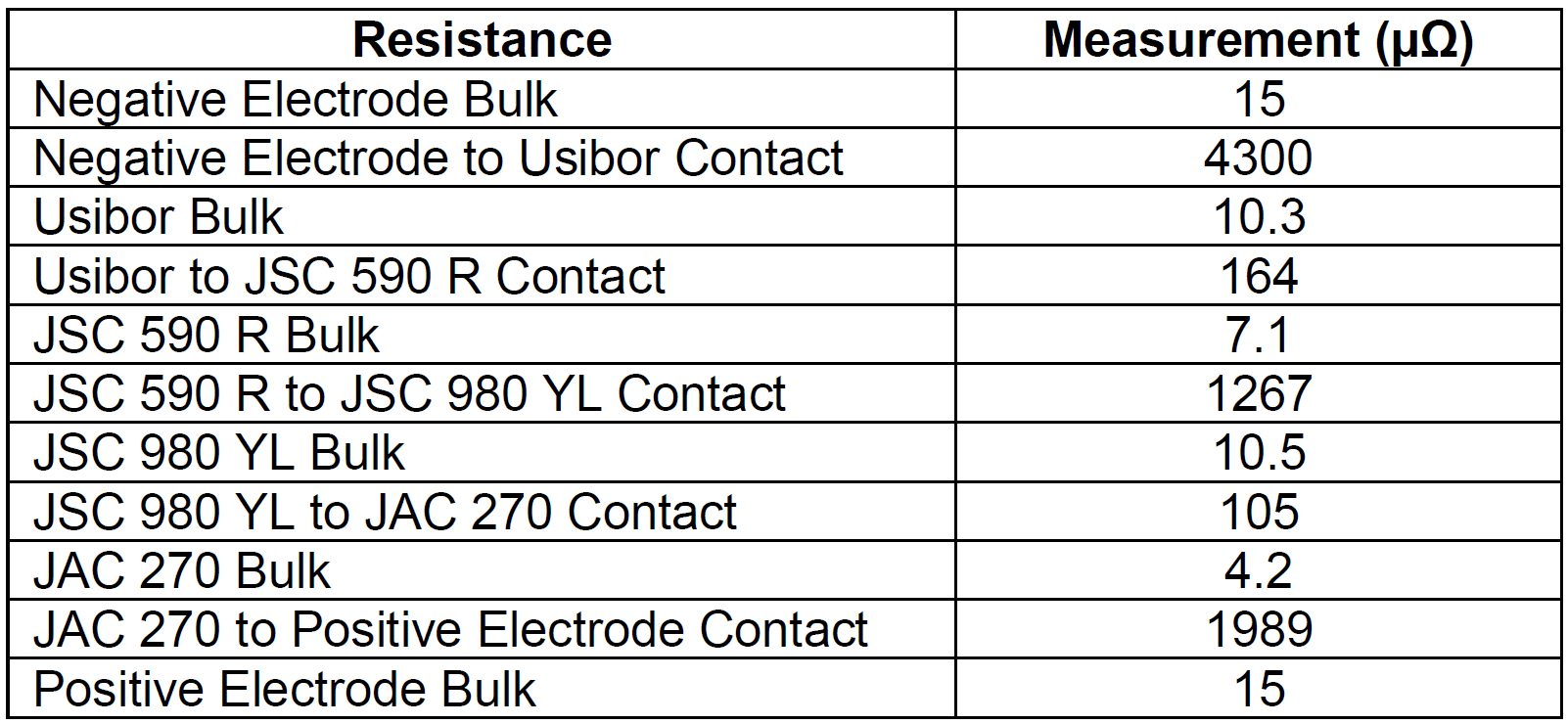
Table 3: Resistance Measurements of Weld Materials and Weld Stack Interfaces.N-11
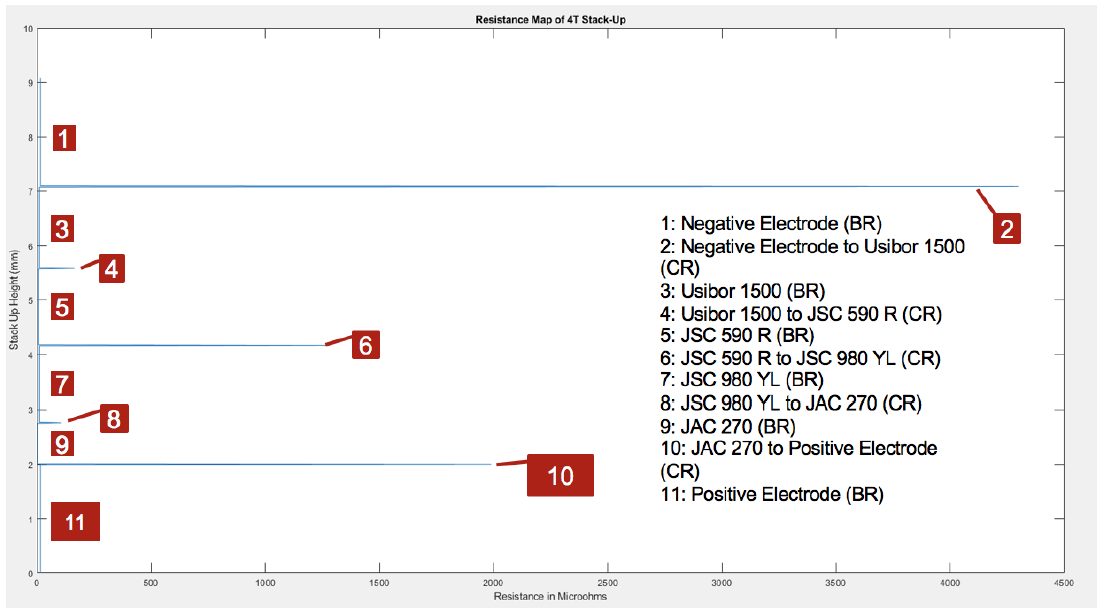
Figure 5: Resistance Graph of Weld Stack-Up.N-11
The weld force used was 2.3 kN and the current reduction values are listed in Table 4. Figure 6 and Figure 7 show the still images at each pulse. The heating pattern implies that the JAC270 is forged into the weld nugget.
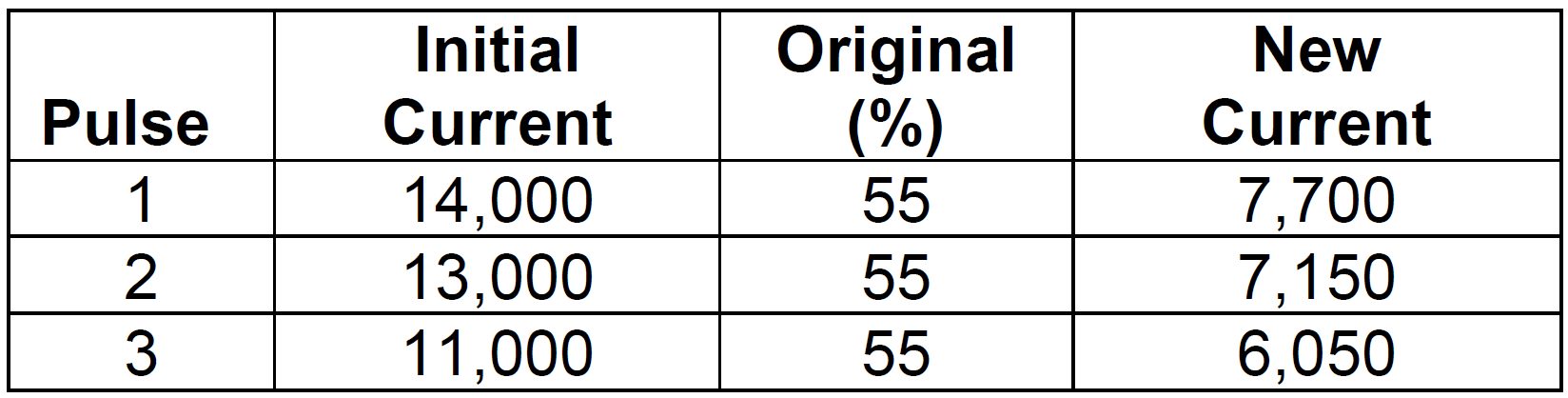
Table 4: Current Reduction for High-Speed Video Welds.N-11
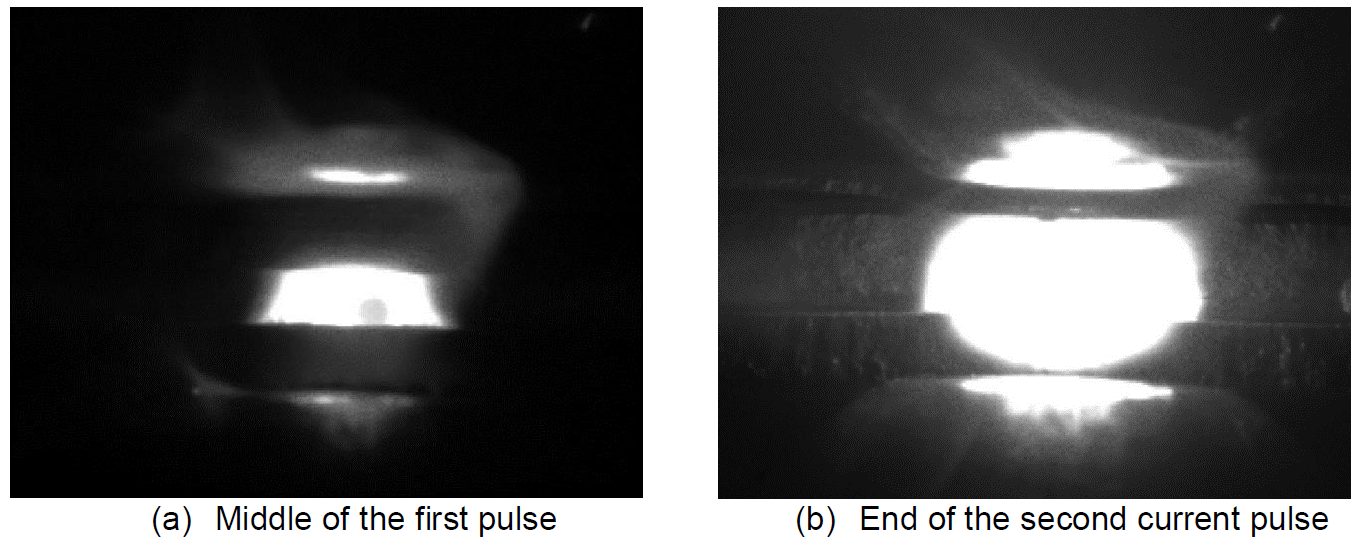
Figure 6: Still Images from High-Speed Video.N-11
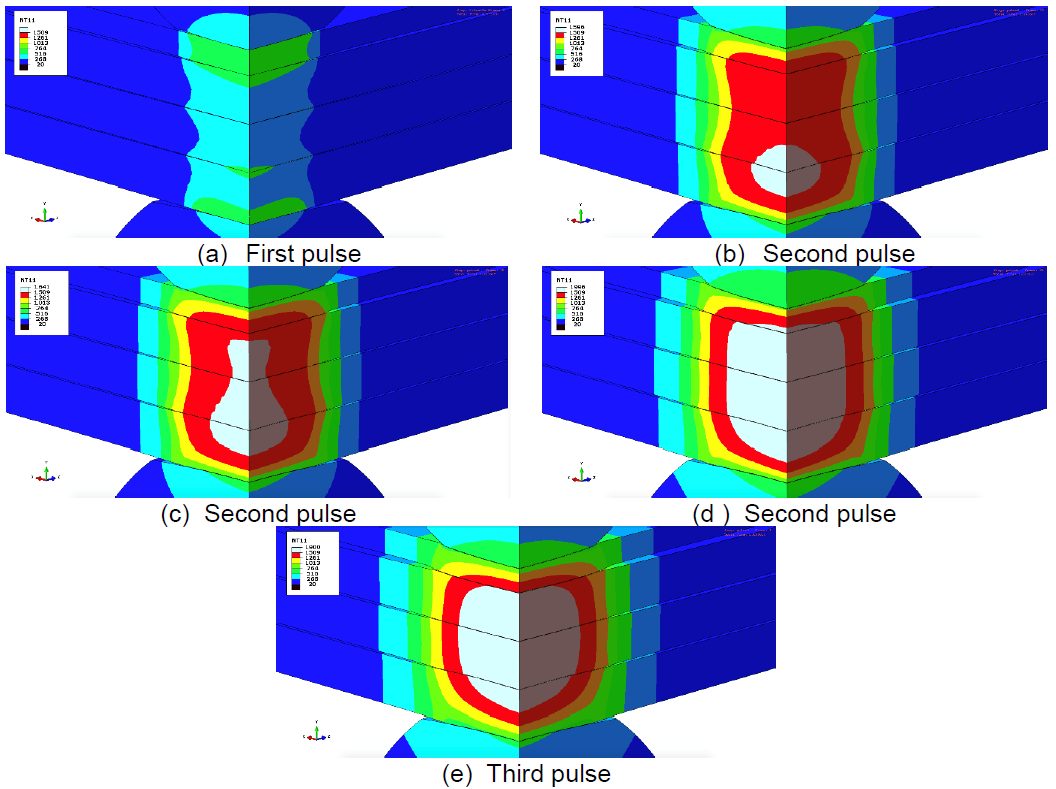
Figure 7: Still Images from Weld Simulation of the Down-Selected Schedule using 8- and 6-mm Class 1 Electrodes.L-58
RSW of Dissimilar Steel
This article is the summary of a paper entitled, “HAZ Softening of RSW of 3T Dissimilar Steel Stack-up”, Y. Lu., et al.L-15
Electromechanical Model
The study discusses the development of a 3D fully coupled thermo-electromechanical model for RSW of a three sheet (3T) stack-up of dissimilar steels. Figure 1 schematically shows the stack-up used in the study. The stack-up chosen is representative of the complex stack-ups used in BIW. Table 1 summarizes the nominal compositions of the three steels labeled in Figure 1.
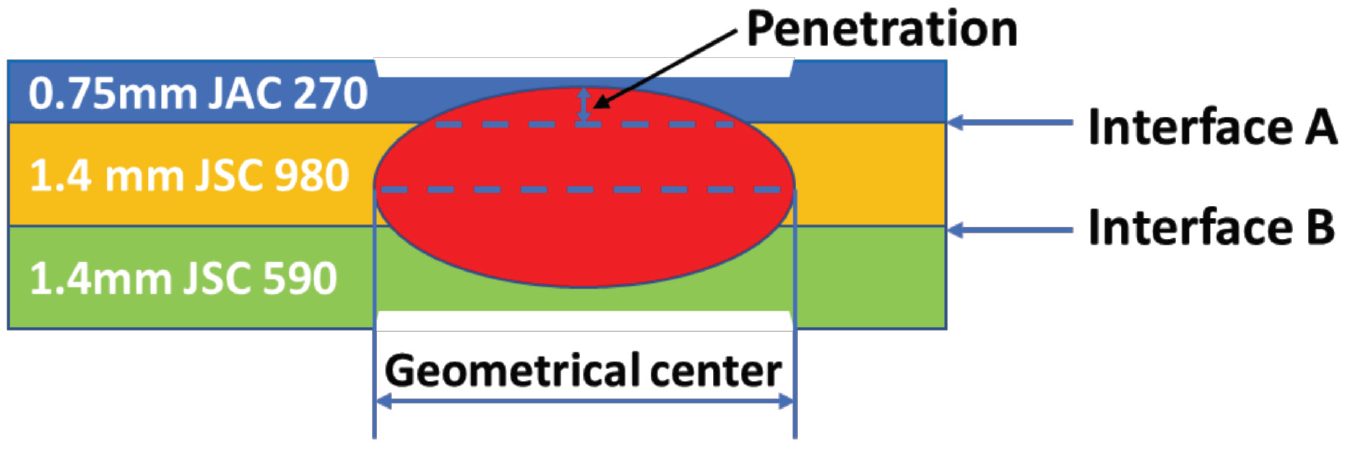
Figure 1: Schematics of the 3T stack-up of 0.75-mm-thick JAC 270/1.4-mm-thick JSC 980/1.4-mm-thick JSC 590 steels.L-15

Table 1: Nominal Composition of Steels.L-15
JAC270 is a cold rolled Mild steel with a galvanneal coating having a minimum tensile strength of 270 MPa. JSC590 and JSC980 are bare cold rolled Dual Phase steels with a minimum tensile strength of 590 MPa and 980 MPa, respectively.
The electrodes used were CuZr dome-radius electrodes with a surface diameter of 6 mm. The welding parameters are listed in Table 2.
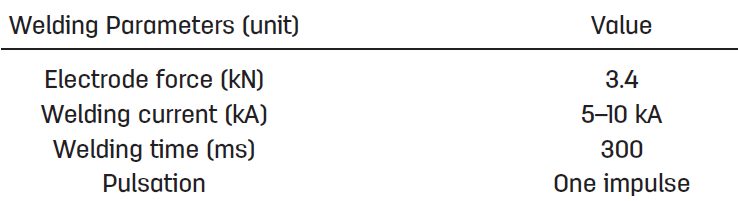
Table 2: Welding Parameters for Resistance Spot Welding of 3T Stack-Up of Steel Sheets.L-15
Figure 2 shows consistent nugget dimensions between simulation and experiment, supporting the validity of the RSW process model for 3T stack-up. The effect of welding current on nugget penetration into the thin sheet is similar to that on the nugget size. It increases rapidly at low welding current and saturates to 32% when the welding current is higher than 9 kA, as shown in Figure 2C.
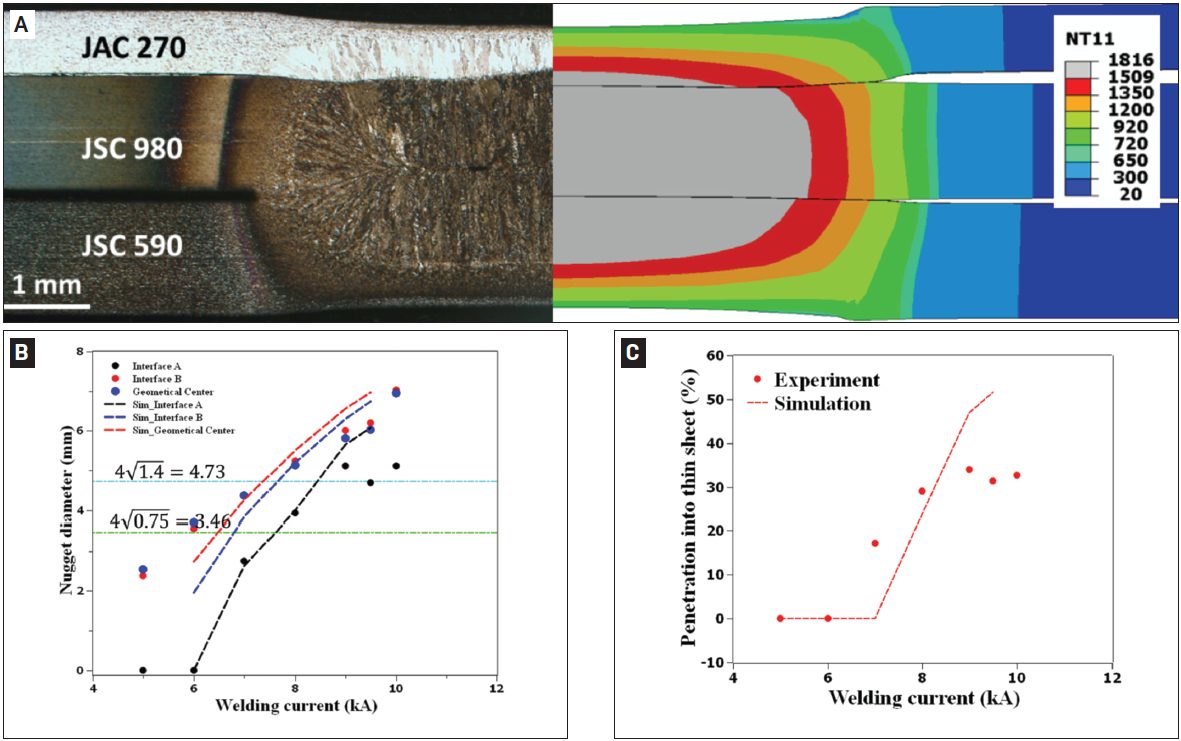
Figure 2: Comparison between experimental and simulated results: A) Nugget geometry at 8 kA; B) nugget diameters; C) nugget penetration into the thin sheet as a function of welding current. In Figure 2A, the simulated nugget geometry is represented by the distribution of peak temperature (in Celsius). The two horizontal lines in Figure 2B represent the minimal nugget diameter at Interfaces A and B calculated, according to AWS D8.1M: 2007, Specification for Automotive Weld Quality Resistance Spot Welding of Steel. Due to limited number of samples available for testing, the variability in nugget dimensions at each welding current was not measuredL-15.
The results for nugget formation during RSW of the 3T stack-up are show in Figures 3-5. Figure 2 shows that, at the start of welding, the contact pressure at interface A (thin/thick) has a higher peak and drops more quickly along the radial direction than that at interface B (thick/thick). Due to the more localized contact area (Figure 3), a high current density can be observed at interface A, as shown in Figure 4A. Additionally, due to the high current density at interface A, localized heating is generated at this interface, as shown in Figure 5A.
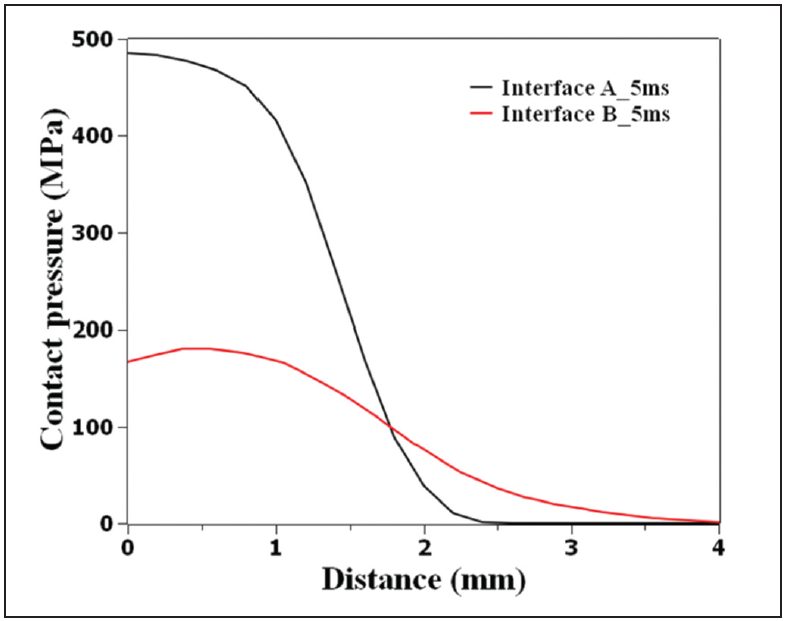
Figure 3: Calculated contact pressure distribution at interfaces A (thin/thick) and B (thick/thick) at a welding time of 5 ms, current of 8 kA, and electrode force of 3.4 L-15
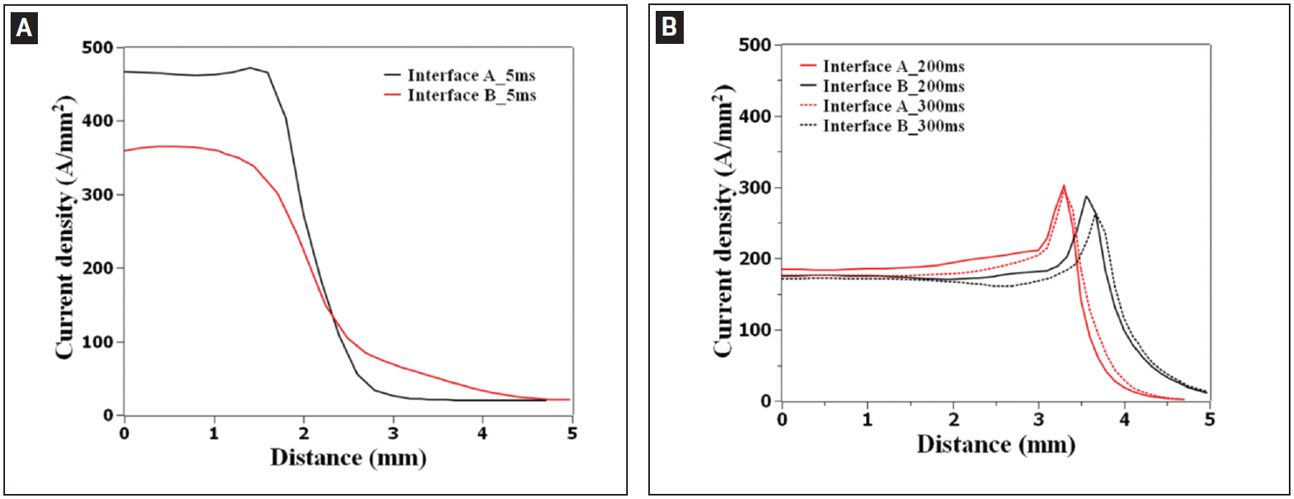
Figure 4: Calculated current density distribution at interfaces A (thin/thick) and B (thick/thick) at welding time of A — 5 ms; B — 200 and 300 ms.L-15
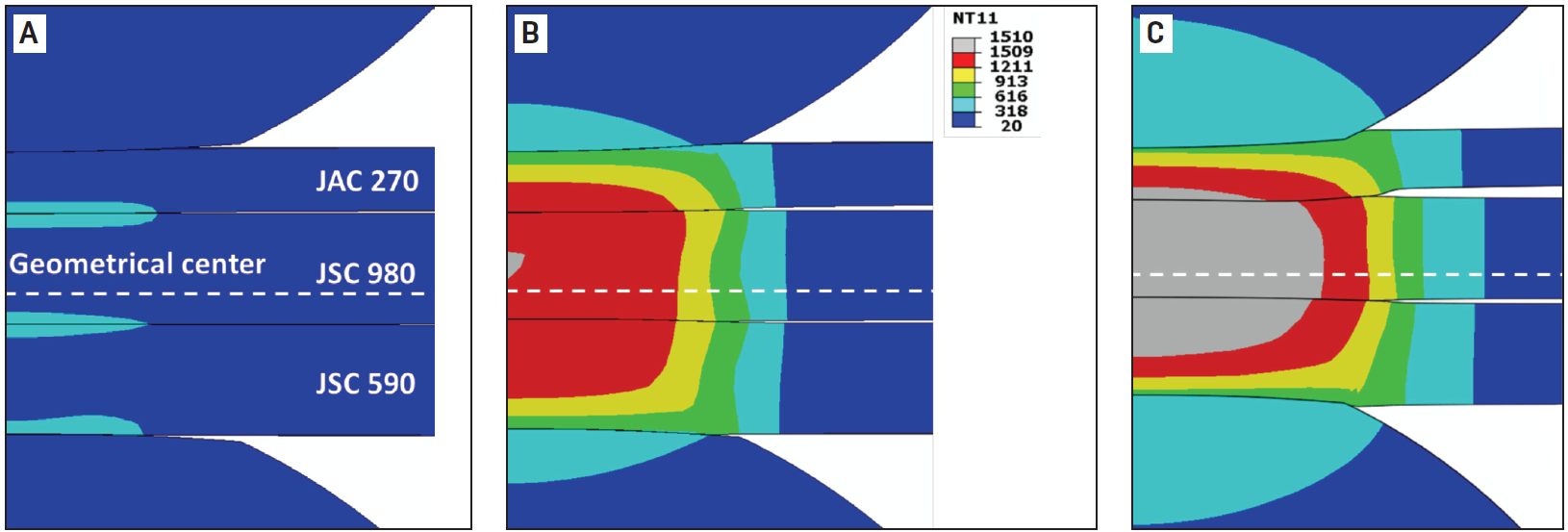
Figure 5: Temperature distribution during resistance spot welding of 3T stack-up at welding times of A) 5 ms; B) 102 ms; C) 300 ms. Welding current is 8 kA and electrode force is 3.4 kN. Calculated temperature is given in Celsius.L-15
As welding time increases, the contact area is expanded, resulting in a decrease of current density. The heat generation rate is shifted from interfaces to the bulk and the peak temperature occurs near the geometrical center of the stack-up.
Figure 6 illustrates that the predicted value corresponds well with the experimental data indicating a sound fitting to the isothermal tempering experimental data.
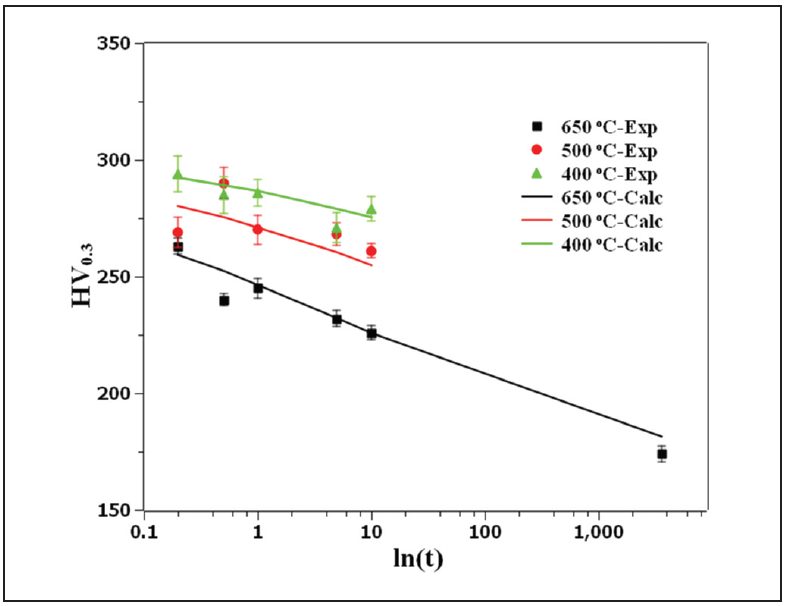
Figure 6: Comparison of the measured hardness with JMAK calculation showing the goodness of fit of the JSC 980 tempering kinetics parameters.L-15
Figure 7 shows the predicted hardness map of RSW 3T stack-up as well as the predicted and measured hardness profiles for JSC 980.
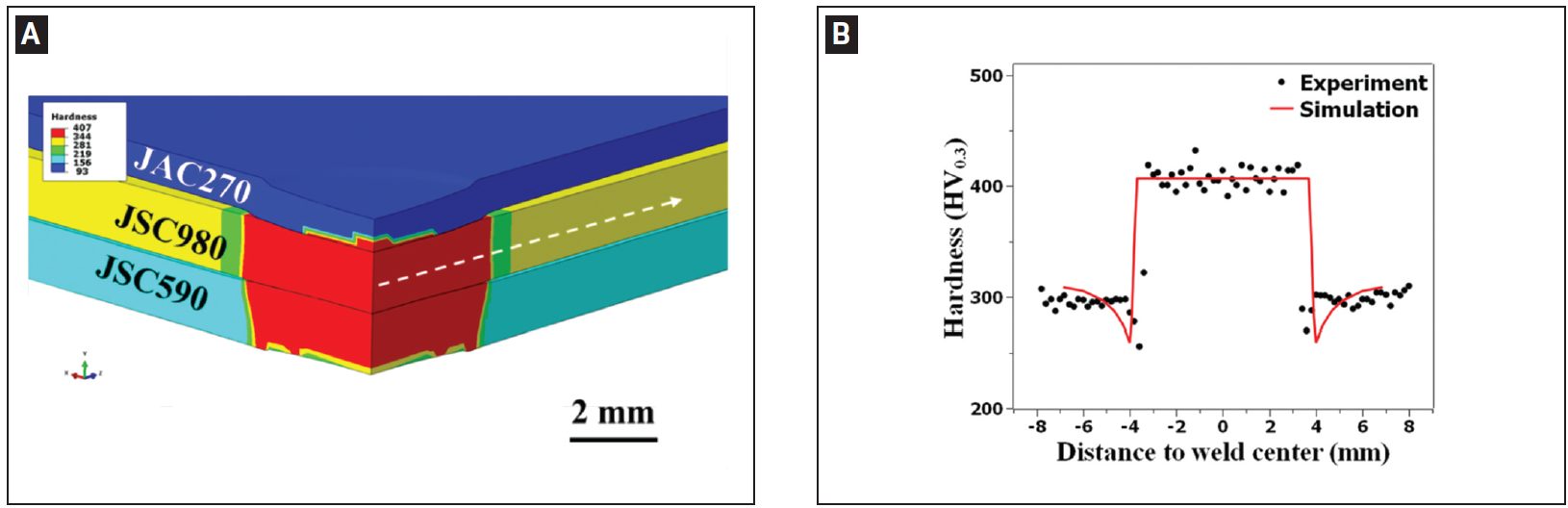
Figure 7: A) Predicted hardness map of resistance spot welded 3T stack-up; B) predicted and measured hardness profiles along the line marked in (A) for JSC 980.L-15
RSW Modelling and Performance
The advantages of numerical simulations for resistance welding are obvious for saving time and reducing costs in product developments and process optimizations. Today’s modeling techniques can predict temperature, microstructure, stress, and hardness distribution in the weld and Heat Affected Zone (HAZ) after welding. Commercial modeling software is available which considers material type, various current modes, machine characteristics, electrode geometry, etc. An example of process simulation results for spot welding of 0.8-mm DC 06 low-carbon steel to 1.2-mm DP 600 steel is shown in Figure 1. Obviously, this technique can apply to dissimilar thicknesses, material types, and geometries. Application of adhesives is also being used with these simulations. This simulation techniques are found to be very beneficial to predict vehicle crashworthiness as it can dramatically reduce the cost of crash evaluations.
You will find several articles in this section describing RSW modelling studies and procedures.
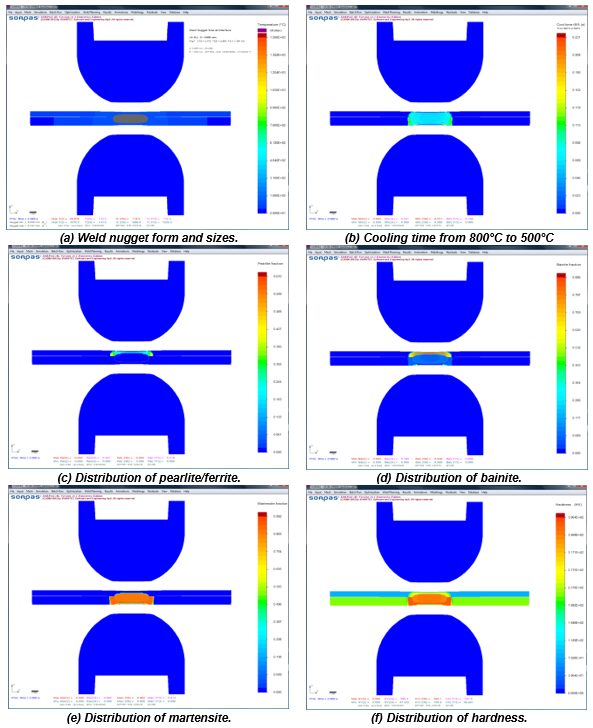
Figure 1: Simulation results with microstructures and hardness distribution for spot welding of 0.8-mm DC06 low-carbon steel to 1.2-mm DP 600 steel.Z-1