topofpage
- Tool Materials
- Surface Hardening Treatments and Coatings
- Stamping Tool Failure Modes
- Tool Steels Production Routes
- Tool and Die Considerations When Working With Higher Strength Steels
- Case Study: Tooling Influences Cut Edge Quality
- Case Study: Upgraded Tool Steels for Upgraded Sheet Metal Forming
- Key Points
Tool and die wear occurs due to the friction produced from the contact between the sheet metal and the tooling surface. Damage to the die surface can cause a gradual loss of tooling material, and scoring or burnishing damage to the sheet metal surface may be stress risers leading to premature failure in formed parts.
Impacting tool wear are the die material, strength of sheet metal, contact pressure, surface finish of the sheet and tooling, sliding velocity, temperature, coating of the die, and lubrication used. Advanced steel grades, where work hardening during stamping further increases the strength of an already high strength product, may result in additional die wear. Die wear beyond a critical point calls for replacement of the current die, impacting turnaround times and to production losses.
New die materials and better die coatings exist which minimize the impact of excessive tooling wear when forming AHSS. These new die materials include wrought and cast tool steels as well as powder metallurgy tool steels, which retain hardness without compromising the toughness of the material. Furthermore, hard material coatings and nitriding can improve the tribological properties of die surfaces.
Tool Materials
Most tool materials for sheet metal forming are cast iron, cast steel, or tool steels.N-12 Cast iron grades used for stamping applications are gray cast irons (like G2500, G25HP and G3500) and pearlitic ductile irons (D4512, D6510, and D7003, among others). Cast steel grades include S0030, S0050A, S7140, and S2333. Tool steels include TD2 (a high wear / low shock resistant tool steel), TS7 (a high shock / low wear resistant tool steel) and TA2 (a balanced medium wear / medium shock resistant tool steel). These designations come from Reference NAAMS, with other designations in Citations A-37, A-38, I-11, J-7, J-8, J-9 Many of these designations overlap and represent the same or highly similar product. For example, ASTM A681 D2, JIS G4404 SKD11, and ISO 4957 X153CrMoV12 cover the same alloy tool steel.
In general, existing tool and die shop procedures to select the appropriate die material are applicable to select dies made to stamp Advanced High Strength Steels. However, the considerably higher strength level of these grades exerts proportionally increased load on the die material. AHSS grades might reach hardness values 4 to 5 times higher than mild steel grades. This is partially due to the microstructure of the sheet metal itself since some grades achieve higher strength from the microstructural phase martensite. Some martensitic grades (MS) have a tensile strength higher than 2000 MPa. This strength level corresponds to Rockwell C values higher than 57, meaning that the sheet metal hardness is approaching the tooling hardness.
The higher forces required to form AHSS require increased attention to tool specifications. The three primary areas are:
- Stiffness and toughness of the tool substrate for failure protection.
- Harder tool surface finishes for wear protection.
- Surface roughness of the tool.
The accepted amount of wear/galling between maintenance periods is a key factor in determining the performance requirements of draw dies, punches, and other tooling components. Some of the key elements that affect the die material specification include:
- The chosen sheet metal to be processed, as characterized by strength, thickness, surface coating, and surface profile (roughness and peak count).
- Die construction, machinability, radii sharpness, surface finish, and die hardness, specifically on draw beads and radii.
- Lubrication.
- Targeted cost per part.
Counteracting the increased applied load required to form AHSS grades is a potential reduction in sheet thickness. This thickness reduction leading to lighter weight parts is one of the key drivers promoting expanded use of Advanced High Strength Steels. Unfortunately, the reduced thickness of the steel increases the tendency to wrinkle. Suppressing these wrinkles requires higher blankholder forces. Any formation of wrinkles will increase the local load and accelerate the wear effects. Figure 1 shows a draw die with severe die wear due to excessive wrinkling on a DP980 part. It is not uncommon to replace these high wear areas with a more durable tool steel insert to minimize this type of excessive wear condition.
Figure 1: Draw die with significant wear due to excessive wrinkling on a DP980 part. S-45
Surface Hardening Treatments and Coatings
Surface treatments and coatings help increase tool life and reduce friction. Flame or induction hardening heat treatments, nitriding, and chrome plating are common surface treatment techniques used. However, each of these can fail under the high contact pressure that is present when stamping advanced high strength steels. Coating the inserts adds additional wear and friction benefits.
Many surface hardening options exist which improve the wear resistance.A-7 Carbon content limits the achievable surface hardness with either flame hardening or induction hardening. Tools hardened with either approach must be quenched after heating, which increases the risk of distortion. Laser beam hardening relies on the high thermal conductivity of underlying base tool steel to self-quench, which reduces the magnitude of distortion. Further minimizing distortion: the energy input in laser beam hardening is approximately 10% of flame hardening.
Carbon and nitrogen increase the strength and hardness of sheet steels. Similarly, carburizing and nitriding tool steels create a hard, wear-resistant surface layer. Carburizing is done at a higher temperature, which carries the risk of distortion. Nitriding takes primarily one of two forms: gas nitriding and plasma (ion) nitriding. Ion nitriding is faster than gas nitriding, accomplished at a lower processing temperature, and minimizes the thickness of the brittle “white layer.” A-39
Chrome plating of tools and dies has been an option to increase wear resistance, but may exhibit microcracking. Environmental concerns further limit its use. In addition, studies show that is it not the best option for tools used to form advanced high strength steels.Y-6
A high hardness, low friction coating results in a wear resistant surface that lowers the risk for galling. Coatings include titanium nitride (TiN), titanium carbide (TiC), titanium carbonitride (TiCN), titanium aluminum nitride (TiAlN) and chromium nitride (CrN). Common application methods are physical vapor deposition (PVD), chemical vapor deposition (CVD), and thermal diffusion (TD).
The strength of metallurgical bonds produced in the CVD and TD processes are greater than physical bond associated with the PVD approach. However, application of CVD and TD coatings occurs at around 1000 degrees C, which is likely in the austenite region of the tool steel. This high temperature can soften the die, which then necessitates a subsequent rehardening process, and may also cause dimensional distortion. For these reasons, several global automakers specify only PVD coatings.
The benefits of PVD coatings in reducing galling are apparent in Figure 2, which compares a cut edge after blanking of 200,000 parts of CR 500Y/800T-DP. Use of cutting steels with a PVD-applied TiAlN coating results in a cleaner, more uniform edge.
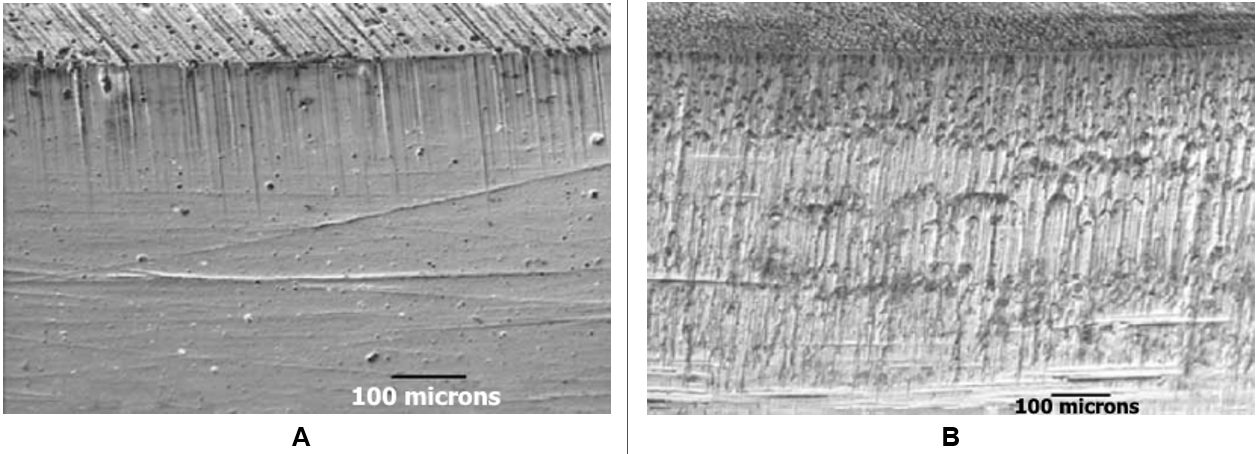
Figure 2: PVD-applied TiAlN-coated cutting steel (image A) reduces galling compared with an uncoated cutting steel (image B). Edges are shown after 200,000 parts produced from CR 500Y/800T-DP. T-20
Since coatings may crack, it is important that the substrate has sufficient hardness/strength to avoid even the slightest plastic deformation of the tool surface. Therefore, the recommended practice is to perform an initial surface hardening treatment, typically flame or induction hardening followed by ion nitriding, to develop substrate hardness and strength before applying the coating. Surface roughness must be as low as possible before coating, with average surface roughness (Ra) values below 0.2 μm recommended. This roughness level approximates a 600-grit sandpaper finish.
Considering the high cost of coated tool steels, a recommended approach is to construct large forming tools from relatively inexpensive and soft materials, such as cast iron or low-grade tool steel. Locations subject to severe wear are candidates for inserts of high-grade tool steels with an appropriate coating engineered for the application.
Ceramic tool inserts have extreme hardness for wear resistance, high heat resistance, and optimum tribological behavior, but have poor machinability and severe brittleness. Potentially offsetting the higher cost are reduced maintenance and increased productivity. While not commonly used, the ceramic tool inserts offer a possible solution to high interface loading and wear.
Select tool steel inserts for forming dies according to the sheet metal and the forming severity. These inserts should have a surface coating when processing DP 350/600 and higher grades. Initial tryout should be completed before coating, so that die adjustments and springback compensation efforts do not lead to removal of the newly applied coating. Allow for tooling recuts during this tryout loop to ensure the resulting tool has sufficient mass and stiffness. Different friction and metal flow conditions should be expected between the initially uncoated and the ultimately coated tool steels.
The optimal surface treatment may increase upfront cost, but will reduce the rework and die maintenance cost over the life of the die. Shown in the top two lines of Figure 3 are the benefits of plasma ion nitriding a flame hardened graphite-bearing cast iron tool (GGG70L) when forming 1 mm thick electrogalvanized dual phase steel. The bottom two lines show the effect on AISI D2 (DIN 1.2379 or JIS SKD11), highlighting only minimal tooling wear over the 5000 parts evaluated.
Figure 3: Plasma ion nitriding leads to reduced tool wear. GGG70L is a flame hardened spheroidal graphite-bearing cast iron and DIN 1.2379 is AISI D2 or JIS SKD11 cold work tool steel.T-11
Figure 4 compares the surface appearance of the same tool steel with different coatings. On the left is a chrome-plated tool which exhibited adhesive and abrasive wear, and ran for only 50,000 parts. Shown on the right is an ion nitrided tool steel which was chromium nitride coated using PVD, and produced more than 1.2 million parts.
Figure 4: Tool steel surface. Left image: Chrome plated, failed after 50,000 parts; Right image: Ion nitride tool steel, chromium nitride PVD coating, produced more than 1.2 million parts. J-10
Heat produced from stamping AHSS grades interacts with the tool material and coating, which may impact friction and metal flow. 1mm thick hot dip galvannealed CR340Y/590T-DP-GA was evaluated in a laboratory set-up.S-46 Initially at room temperature, die surface temperature increased to 65 °C (150 °F) after 10 cycles of passing this DP590 grade across a tool radius and significant zinc powdering occurred. Less powdering occurred with the use of a die coolant. Cooling the dies also helped to reduce the surface scoring and associated friction [Figure 5].
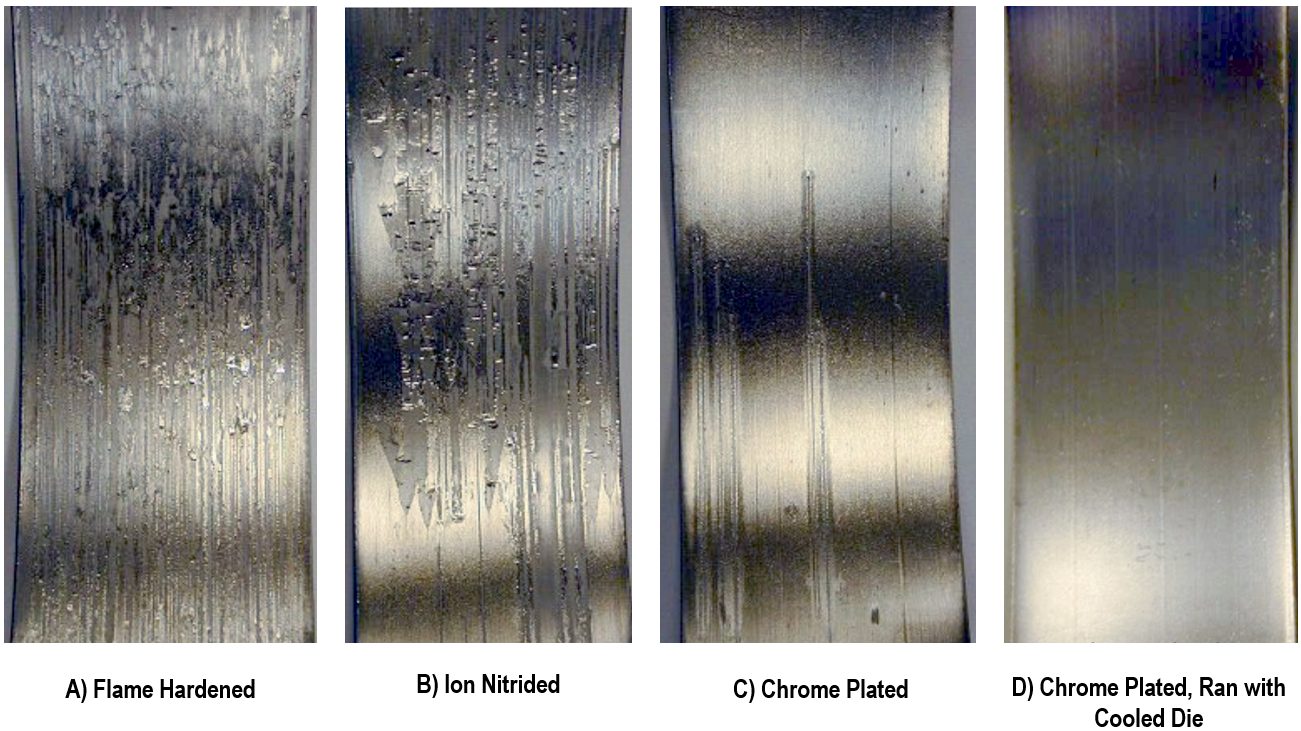
Figure 5: Coatings and Die Coolant on D2 Tool Steel Reduce Scoring and Friction with galvannealed dual phase steel. A) Flame Hardened; B) Ion Nitrided; C) Chrome Plated; and D) Chrome Plated with die coolant.S-46
Selection of tool steels for cutting, trimming, and punching tools have similar considerations as forming tools. The base tool steel must have excellent chipping and cracking resistance. Coatings will reduce tool wear. Hardening of the substrate prior to coating will minimize failure due to plastic deformation of the substrate. Coatings reduce the severity of the shock wave produced when cutting advanced high strength steels. See the Cutting / Blanking / Shearing / Trimming page for more information.
It is not advisable to use only one tooling solution for all Advanced High-Strength Steels. One study showed that die material and coating methods used in large volume production of steel grades up to and including those with 980 MPa minimum tensile strength were not suitable for forming a grade with 1180 MPa minimum tensile strength.W-18 Furthermore, this study recommends avoiding die materials such as ductile iron and low alloy cast steel when stamping 1180 grade steels.
In addition to selecting the correct die material, it must be processed appropriately. Figure 6 shows the effects of proper heat treatment when stamping a dual phase steel with 980MPa minimum tensile strength.S-45 This same study showed that a PVD coated tool performed best when forming a DP steel without a galvanized coating, yet the PVD coating led to significant zinc buildup when forming a galvanized DP steel. An ion nitride tool coating worked best for galvanized steels.
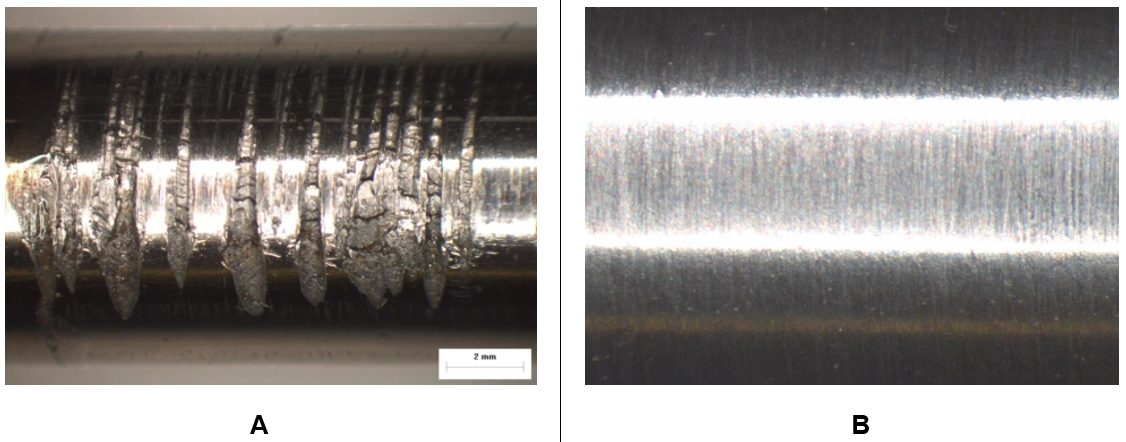
Figure 6: Effect of Heat Treatment on Tools to Stamp Dual Phase Steel. Image A) No heat treatment leads to wear; Image B) Proper heat treatment results in a surface without damage.S-45
Stamping Tool Failure Modes
There are five main types of cold work failure modes involving tool steels – wear, plastic deformation, chipping, cracking, and galling. There is also interaction between these failure modes. Figure 7 shows examples of these failure modes.T-20, U-7
Wear is damage to the tooling surface resulting in material loss and is related to the tooling material hardness, and the type, volume, and distribution of hard particles like oxides or carbides. Wear can also be related to material type and process conditions and involves sliding contact between the tooling and the material. There are two types of wear: abrasive and adhesive.
Abrasive wear occurs when hard particles forced into a surface during the sliding contact leads to removal of metal from the tool steel. Tool steel properties promoting abrasive wear resistance include high hardness of the tool steel and of the carbides, as well as a high volume of large carbides. However, the high hardness targeted for wear resistance makes the material sensitive to notches. Large carbides act as crack initiators, increasing the risk of fatigue cracking.
Adhesive wear occurs with material transfer from one metal surface to another. The friction and heat generated as the sheet metal slides across the tool surface results in micro-welding between the asperities (peaks) on each surface. Failure of these micro-welds occurs with continued relative motion between the two surfaces, with small fragments torn from the weaker side surface and adhering to the other surface. The material ripped out of the tool steel will occasionally stick to the sheet metal surface. With continued metal motion, these pieces may score and damage the tool steel surface resulting in a combination of adhesive and abrasive wear known as mixed wear.
Galling is a physical/chemical adhesion of the sheet metal to the tool surface. The severity of the galling depends on the surface finish and chemical composition of the material and the tool steel and involves the friction and sliding contact between the tooling and the material. Galling, abrasive wear, and adhesive wear are related, and can be minimized through the use of proper surface treatments or coatings on top of a tool steel with high hardness (Figure 8).
Figure 8: Adhesive wear of the tooling leads to abrasive wear scratches on the DP600 sheet surface. Continued abrasive wear leads to galling of the sheet surface. Higher magnification images are shown on the bottom. G-18
Plastic deformation occurs when the stress from contact with the sheet metal exceeds the compressive yield strength of the tool material. A high hardness tool steel helps to avoid this damage.
Chipping occurs when the operating stress levels exceed the fatigue strength of the tool steel, typically found at sharp edges. Microcracks initiate in the high contact area of the tool surface, propagate, and ultimately result in pieces chipping out along edges or at corners. Chipping may initiate in areas affected by adhesive wear. Here, microcracks can nucleate, deepen, and spread, leading to a fatigue failure. A tool steel with high ductility has good chipping resistance, since microcrack initiation and propagation are more difficult.
Cracking occurs when the operating stress levels exceed the fracture toughness of the tool material. Crack formation occurs in the presence of stress concentrators, like grinding and machining marks or design features such as sharp corners or radii. Once the crack forms, unstable crack propagation leads to failure. Microstructural toughness promotes good cracking resistance, as does low hardness. However, low hardness has a detrimental effect on the resistance to the other failure mechanisms and is not normally a good solution.
Higher strength steels lead to greater demands on the wear resistance and mechanical strength of the tool material. Forming operations require high wear and galling resistance and compressive strength. Cutting operation require a combination of high wear resistance, high galling resistance, high compressive strength, high chipping, and total cracking resistance.
Tool Steels Production Routes
Tool materials must balance compressive strength and toughness with resistance to wear, thermal, and mechanical stresses.
Conventional highly alloyed tool steels are produced from large ingots. The slow solidification leads to microstructural segregation, forming large carbide networks which turn into carbide stringers after processing. These networks are beneficial for wear resistance, but reduces fatigue strength and toughness.
Alternate approaches minimizing segregation reduces these concerns. Two such production methods are electroslag remelting and powder metallurgy.T-20
Electroslag remelting (also known as electroslag refining, ESR) is a progressive melting process used to produce porosity-free ingots of uniform chemistry. Under a protective atmosphere, only a small portion of the ingot is liquid at any one time, and solidification occurs in a controlled manner. This processing approach results in tool steels with increased cleanliness, smaller carbides, and improved ductility and fatigue properties. It is relatively expensive, leading to its use in some specialized tool steel applications.
Rather than slowly solidifying in a large ingot, powder metallurgy (PM) production involves first atomizing a stream of molten metal using a high pressure inert gas, resulting in droplets that rapidly solidify into powder. Segregation is typically a fraction of the powder diameter, which is on the order of 100 μm. Hot isostatic pressing (HIPing) consolidates the collected powders, which is subsequently rolled or forged in a similar manner used for ingots.
Without the concern of macro-segregation or large carbides, the PM approach allows for manufacturing of more highly alloyed tool steels than is possible with conventional ingot metallurgy. Here, the carbides are smaller and more evenly distributed even compared with the ESR approach, leading to a balance of wear resistance and fatigue life. PM tool steels have enhanced resistance to abrasive wear, adhesive wear, chipping, and cracking. Coatings improve galling resistance.
Tool and Die Considerations When Working With Higher Strength Steels (U-13)
Metal stampers and die shops experienced with mild and HSLA steels often have problems making parts from AHSS grades. The higher initial yield strengths and increased work hardening of these steels can require as much as four times the working loads of mild steel. Some AHSS grades also have hardness levels approaching the dies used to form them.
The higher stresses required to penetrate higher-strength materials require increased punch-to-die clearances compared to mild steels and HSLA grades. Why? This clearance acts as leverage to bend and break the slug out of the sheet metal. Stronger materials need longer levers to bend the slug. The required clearance is a function of the steel grade and tensile strength, and sheet thickness.
Increasing cutting clearance can result in punch cracking and head breakage due to higher snapthrough loads and reverse-unloading forces within the die. Adding shear angles to the punch face helps reduce punch forces and reverse unloading.
Tight cutting clearances increase the tendency for die galling and chipping. The severity of galling depends on the surface finish and microstructure of both the tool steel and work material. Chipping can occur when process stresses are high enough to cause low-cycle fatigue of the tooling material, indicating that the material lacks toughness.
Tempering of tools and dies represents a critical heat-treatment step and serves more than one purpose, but of primary concern is the need to relieve residual stresses and impart toughness. Dies placed in service without proper tempering likely will experience early failure.
Dies made from the higher-alloy tool-steel grades (D, M or T grades) require more than one tempering step. These grades contain large amounts of retained austenite and untempered martensite after the first tempering step and require at least one more temper to relieve internal stresses, and sometimes a third temper for even greater toughness.
Unfortunately, heat treatment remains a “black-box” process for most die shops and manufacturing companies, who send soft die details to the local heat treat facility, with hardened details returned. A cursory Rockwell hardness test may be conducted at the die shop when the parts return. If they meet hardness requirements, the parts usually are accepted, regardless of how they may have been processed—a problem, as hardness alone does not adequately measure impact toughness.
Case Study: Tooling Influences Cut Edge Quality
Consider this scenario: An automotive structural part has been in production for years as a conventional high strength steel with a minimum yield strength of 280 MPa, CR280Y350T-LA. In order to meet increasing global safety regulations, the automaker converts the part to a dual phase steel, CR340Y590T-DP. Even though these grades have relatively close minimum yield strength levels as produced at the steel mill, dual phase steels have excellent work hardening characteristics and are bake hardenable. These are among the reasons for their favorable response in crash events in comparison to HSLA grades.
The stamping location attempted to do a direct swap, substituting the DP steel for the HSLA grade with no changes to the part or process. Immediately after the grade change, scrap rates increased significantly. The failures were all determined to be local formability edge fractures; investigation revealed that the edge of the configured blank remained as the final product edge which split during forming and subsequent flanging. Examination of the edge revealed a burr as well as a non-uniform cut edge appearance. The tool showed signs of chipping.
Issues found, along with corrective actions:
- The tool steel used for HSLA (uncoated D2) was not appropriate for stamping DP590. Upgrade to a more durable tool steel with good chipping resistance. Add a surface treatment or coating if needed for additional wear resistance.
- The stamper used the traditional 10% tooling clearance when blanking HSLA. The recommended clearance for DP590 at the thickness studied is 15%.
- The flanging operation further expanded the edge beyond what occurred in the draw die. DP steels work harden to a much greater degree than HSLA steels. As such, stretch flanging a cut edge significantly increases the potential for edge fracture in this stronger product. Adding a metal gainer to the draw die ensures the flanging operation performs only bending and straightening, and does not further stretch a cut edge.
Making these changes to accommodate the new grade eliminated scrap from this process.
Case Study: Upgraded Tool Steels for
Upgraded Sheet Metal Forming (M-20)
Multi-phase steels are complex to cut and form, requiring specific tooling materials. The tooling alloys which have been used for decades, such as D2, A2 or S7, are reaching their load limits and often result in unacceptable tool life. The mechanical properties of the sheet steels achieve tensile strengths of up to 1800 MPa with elongations of up to 40%. Additionally, the tooling alloys are stressed by the work hardening of the material during processing.
The challenge to process AHSS quickly and economically makes it necessary for suppliers to manufacture tooling with an optimal tool steel selection. The following case study illustrates the tooling challenges caused by AHSS and the importance of proper tool steel selection.
A manufacturer of control arms changed production material from a conventional steel to an Advanced High-Strength Steel (AHSS), HR440Y580T-FB, a Ferrite-Bainite grade with a minimum yield strength of 440 MPa and a minimum tensile strength of 580 MPa. However, the tool steels were not also changed to address the increased demands of AHSS, resulting in unacceptable tool life and down time.
According to the certified metal properties, the 4 mm thick FB 600 material introduced into production had a 525 MPa yield strength, 605 MPa tensile strength, and a 20% total elongation. These mechanical properties did not appear to be a significant challenge for the tool steels specified in the existing die standards. But the problems encountered in production revealed serious tool life problems.
To form the FB 600 the manufacturer used D2 steel. D2 was successful for decades in forming applications. This cold work tool steel is used in a wide variety of applications due to its simple heat treatment and its easily adjustable hardness values. In this case, D2 was used at a hardness of RC 58/60.
While tools manufactured from D2 can withstand up to 50,000 load cycles when forming conventional steels, these particular D2 tools failed after only 5,000 – 7,000 cycles during the forming of FB 600. The first problems were detected on a curl station where mechanical overload caused the D2 tools to break catastrophically, as seen in Figure 9 below. Since the breakage was sudden and unforeseeable, each failure of the tools resulted in long changeover times and thus machine downtime.
Figure 9: Breakage seen in control arm curl tool made from D2, leading to premature failure. Conversion to a PM tool steel having higher impact resistance led to 10x increase in tool life.M-20
Since the cause of failure was a mechanical breakage of the tools, a tougher alternative was consequently sought. These alternatives, which included A2 and DC53® (a registered trademark of International Mold Steel) were tested at RC 58-60 and unfortunately showed similar tool life and failures.
Metallurgical analysis indicated that the failure resulted from insufficient impact strength of the tool steel. This was caused by the increased cross-cut that the work-hardened AHSS exerted on the curl. As an alternative material, a cold work steel with a hardness of 58-60, a tensile strength of about 2200 – 2400 MPa and high toughness was sought. These properties could not be achieved with conventional tool steels. The toolmaker used a special particle metallurgy (PM) tool steel to obtain an optimum combination of impact strength, hardness and wear resistance.
Particle metallurgy (PM) tool steels, due to their unique manufacturing process, represent improvements in alloy composition beyond the capabilities of conventional tool steels. Materials with a high alloy content of carbide formers such as chromium, vanadium, molybdenum and tungsten are readily available. The PM melting process ensures that the carbides are especially fine in particle size and evenly distributed (reference Table 1). This process results in a far tougher tool steel compared to conventional melting practices.
The manufacturer selected Z-Tuff PM® to be used at a hardness of RC 58-60. Employing the identical hardness as the conventional cold work steel D2, a significant increase in impact strength (nearly 10X increase as measured by un-notched Charpy impact values) was realized due to the homogeneous microstructure and the more evenly distributed precipitates. This positive effect of the PM material led to a significant increase in tool life. By switching to the PM tool steel, the service life is again at the usual 40,000 – 50,000 load cycles. By using a steel with an optimal combination of properties, the manufacturer eliminated the tool breakage without introducing new problems such as deformation, galling, or premature wear.
AHSS creates tooling demands that challenge the mechanical properties of conventional tool steels. Existing die standards may not be sufficient to achieve consistent and reliable performance for forming, trimming and piercing AHSS. Proper tool steel grade selection is critical to ensuring consistent and reliable tooling performance in AHSS applications. Powder metallurgical tool steels offer a solution for the challenges of AHSS.
Key Points
- Areas seeing higher working loads require improved tool materials and coatings for both failure protection and wear protection.
- The higher initial yield strengths of AHSS, plus the increased work hardening of DP and TRIP steels can increase the working loads by 400% compared to Mild steels.
- Strength levels of some AHSS grades are associated with the same hardness as the tools intended to form them.
- Advanced powder metallurgy (PM) tool materials are appropriate for some AHSS applications.
- The dominant mode of failure of tool steels should be identified and communicated to the tool steel supplier to select the tool steel with the best properties to combat the tool failure mode for a given AHSS grade.
- PVD coated tool steels are appropriate for stamping AHSS grades without a galvanized coating but may result in zinc buildup when forming galvanized steels. Here, an ion nitride coating may be appropriate.
- Complete initial tryout before application of tool coating. Allow for tooling recuts during tryout loop to ensure the resulting tool has sufficient mass and stiffness.
- Proper selection of tool material, surface treatment, and coating leads to a reduction in maintenance, repair, and scrap/rework costs. Further offsetting the upfront tooling costs are improved process uptime, improved quality, and greater consistency.