Resistance Welding Steel to Aluminium
This article summarizes a paper entitled, “Process, Microstructure and Fracture Mode of Thick Stack-Ups of Aluminum Alloy to AHSS Dissimilar Metal Spot Joints”, by Luke Walker, Colleen Hilla, Menachem Kimchi, and Wei Zhang, Department of Materials Science and Engineering, The Ohio State University.W-9
Researchers at The Ohio State University studied the effects of adding a stainless steel (SS) insert to a dissimilar Advanced High-Strength Steel (AHSS) to aluminum (Al) resistance spot weld (RSW). The SS insert was ultrasonically welded to the Al sheet prior to the RSW being performed. The purpose of the SS is to reduce the intermetallic layer that forms when welding steel to aluminum. This process increases the strength and toughness of the weld. In this study, the process is applied to three sheet (3T) stack up that contains one Al sheet and two 1.2 mm thick Press Hardened (PH) 1500 sheets. The joint strength is measured in lap shear testing and the intermetallic thickness/ morphology is studied after cross sectioning the welds.
During the microstructure evaluation it was noted that Al 6022 contained a larger nugget diameter as compared to the Al 5052 welds. A few potential reasons for the hotter welds were proposed including cleanliness of the electrodes, surface oxides, and thickness of the different alloys. The welds on the Al 5052 stack ups were made first on clean electrodes whereas the Al 6022 was made on potentially dirty electrodes that increased the contact resistance. The effects of different surface oxides are not likely given the SS sheet is ultrasonically welded but could still add to the higher heat input in the RSW. The Al 6022 is 0.2 mm thicker, which could increase the bulk resistance and decrease the cooling effect from the electrodes.
The 3T welds likely had much lower strength and toughness due to cracks that formed at the Al-SS insert interface. These can be attributed to an increase in intermetallic compound (IMC) thickness and the residual stress caused by the forge force. The IMC thickness was measured two ways: The first measurement was of the continuous IMC layer and the second was from the Al-Fe interface to the end of the IMC dendrites (Figure 1, 2 and Table 1). The Al 5052 observed the thickest continuous IMC layer but Al 6022 was close to the Al 5052 thickness. This can be attributed to the increased Si content of Al 6022 which has been shown to decrease the growth of Fe-Al intermetallics.
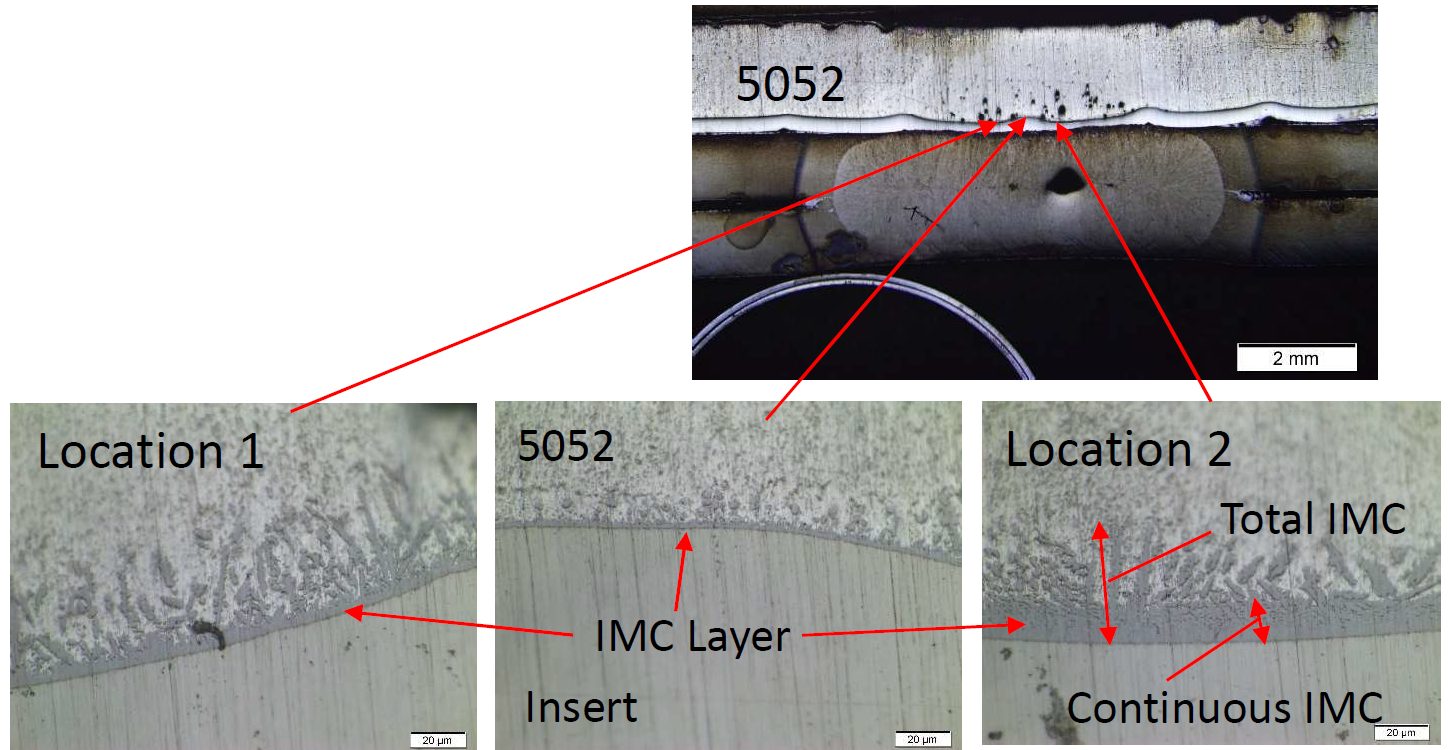
Figure 1: IMC in the Al Alloy 5052 to Stainless-Steel Weld.W-9
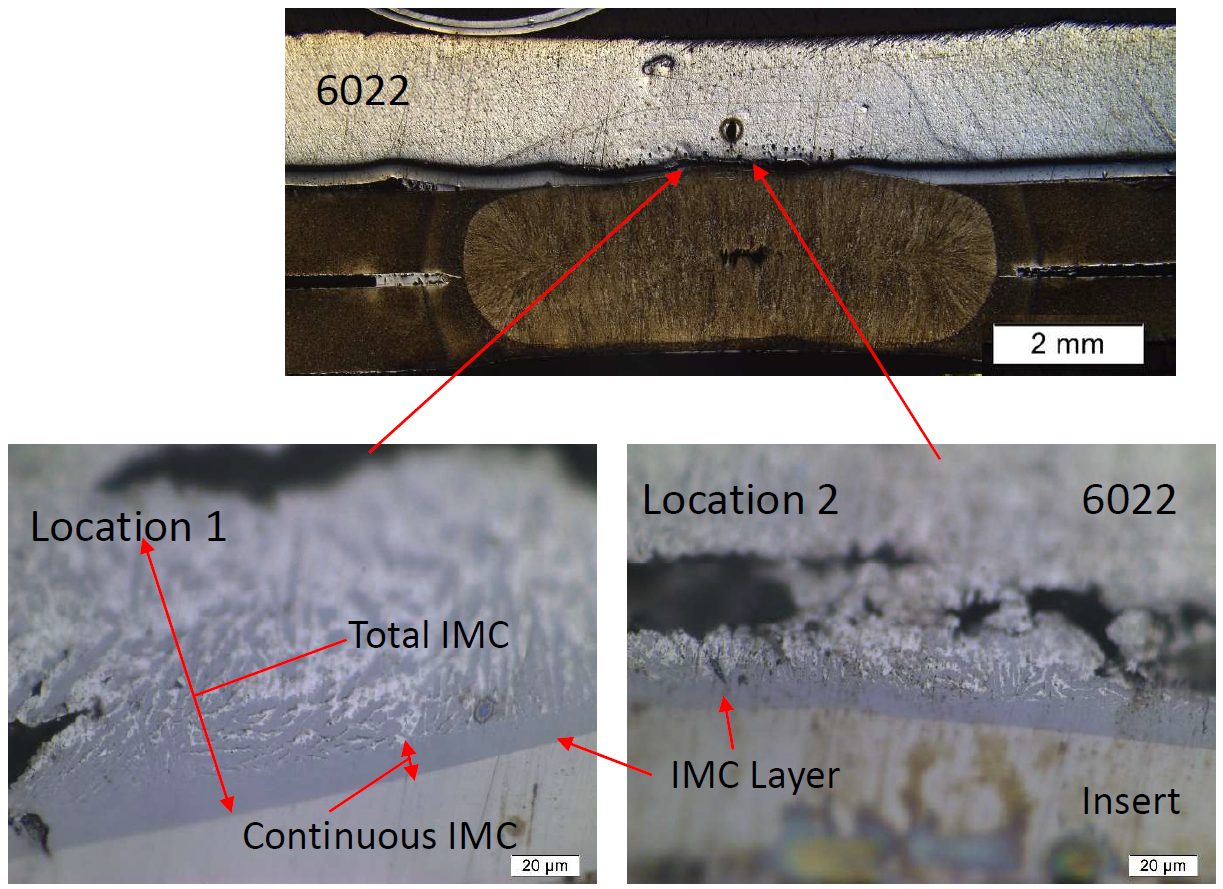
Figure 2: IMC in the Al Alloy 6022 to Stainless-Steel Weld.W-9
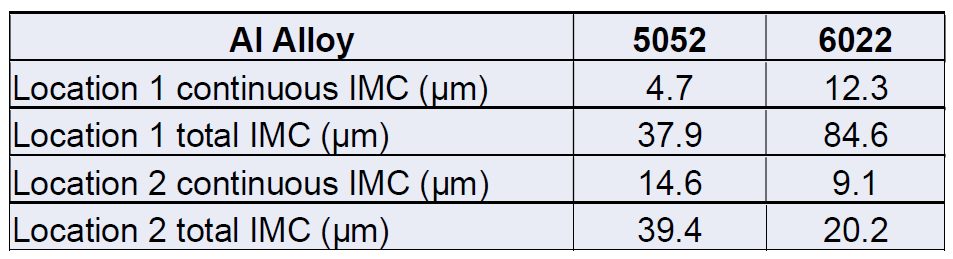
Table 1: IMC Thickness of Both the 5052 Weld and the 6022 Weld.W-9
Referencing Figure 3, the 2T stack-up has a higher tensile strength as well as significantly higher fracture energy absorbed compared to the 3T stack-ups. This is mainly attributed to the failure mode observed in the different stack-ups. The 2T welds had button pullout failure while 3T stack-ups had interfacial Failure.
![Figure 3: Failure Load and Fracture Energy [(A) Al to steel (Al-Us) welds and (B) steel to steel (Us-Us) welds (the 2T 6022 results are from previous work(10))]W-9](https://ahssinsights.org/wp-content/uploads/2021/12/fig4-process.jpg)
Figure 3: Failure Load and Fracture Energy [(A) Al to steel (Al-Us) welds and (B) steel to steel (Us-Us) welds (the 2T 6022 results are from previous work(10))]W-9
The Al 6022 contains higher Si content which likely decreased the growth of the continuous IMC layer but not the overall IMC layer (as seen in Figure 4 and Figure 5) due to higher weld temperatures. The joint strength of the welds in the 3T stack-ups were closer to the expected weld strength unless there was expulsion that caused a 5-kN drop in strength.
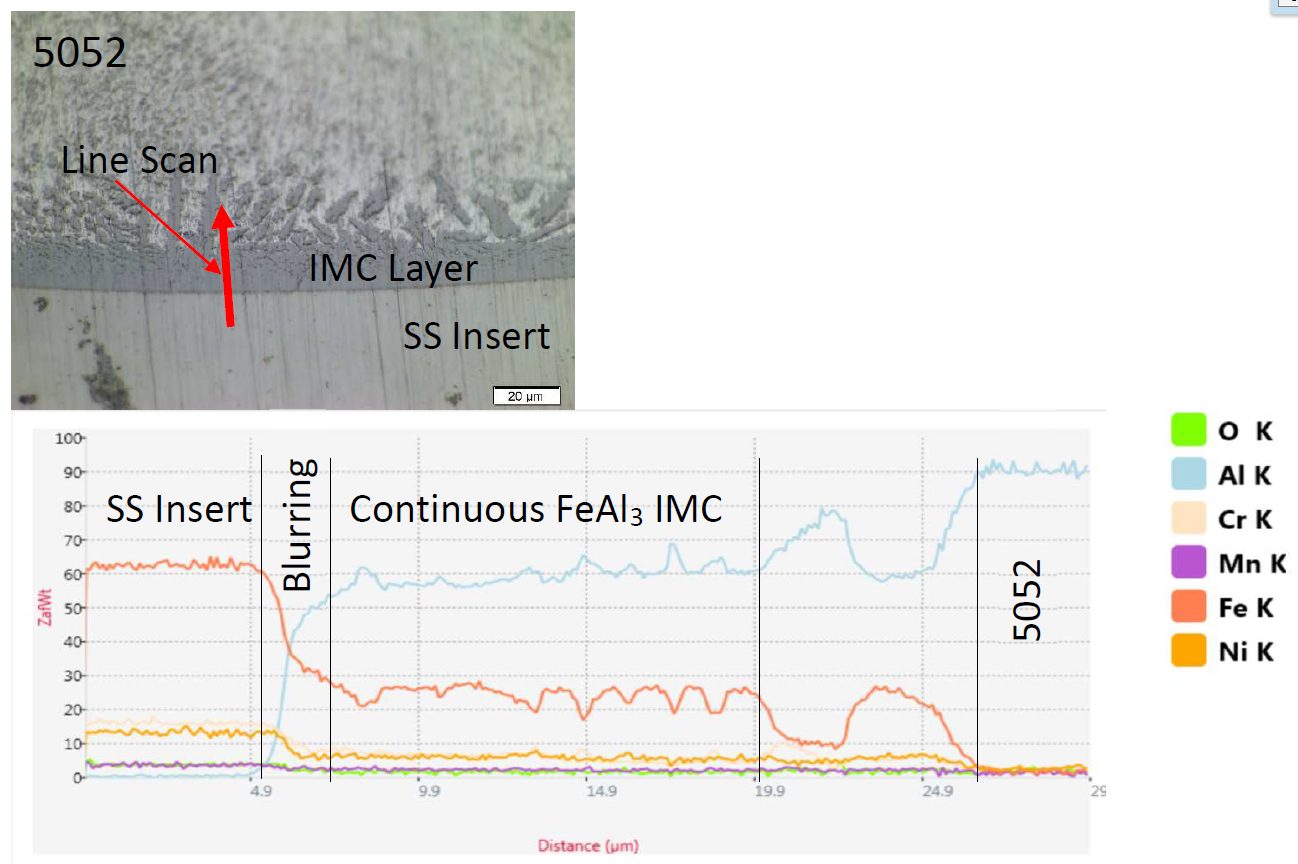
Figure 4: EDS Line Scan of the IMC in Location 2 on the 5052 3T Sample (SS stands for austenitic stainless steel 316).W-9
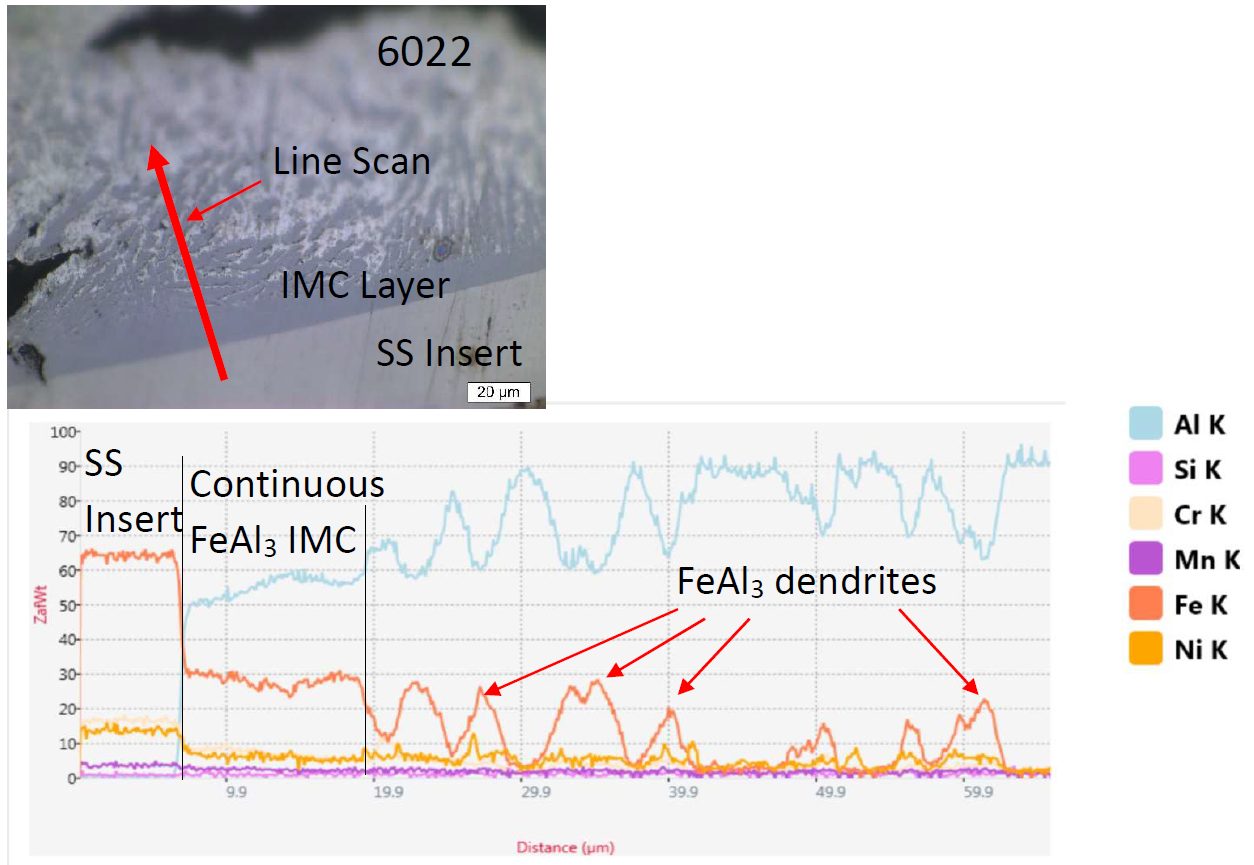
Figure 5: EDS Line Scan of the Intermetallic Layer at Location 1 on the 6022 3T Sample (SS stands for austenitic stainless steel 316).W-9
Joining Dissimilar Materials, Resistance Welding Steel to Aluminium
Recently research at the Ohio State University is developing methods that combine the benefits of ultrasonic welding and resistance spot welding. Y. Lu and co-workersL-10 developed the ultrasonic plus resistance spot welding process (U+RSW). In this process thin aluminium sheet was joined with steel sheet via ultrasonic welding, followed by RSW between the aluminium side of previously sheet and aluminium sheet as shown in Figure 1. In this study they used 1-mm-thick AA6061-T6 to 0.9-mm-thick AISI 1008 steel with 0.4-mm-thick AA6061-T6 as the insert.
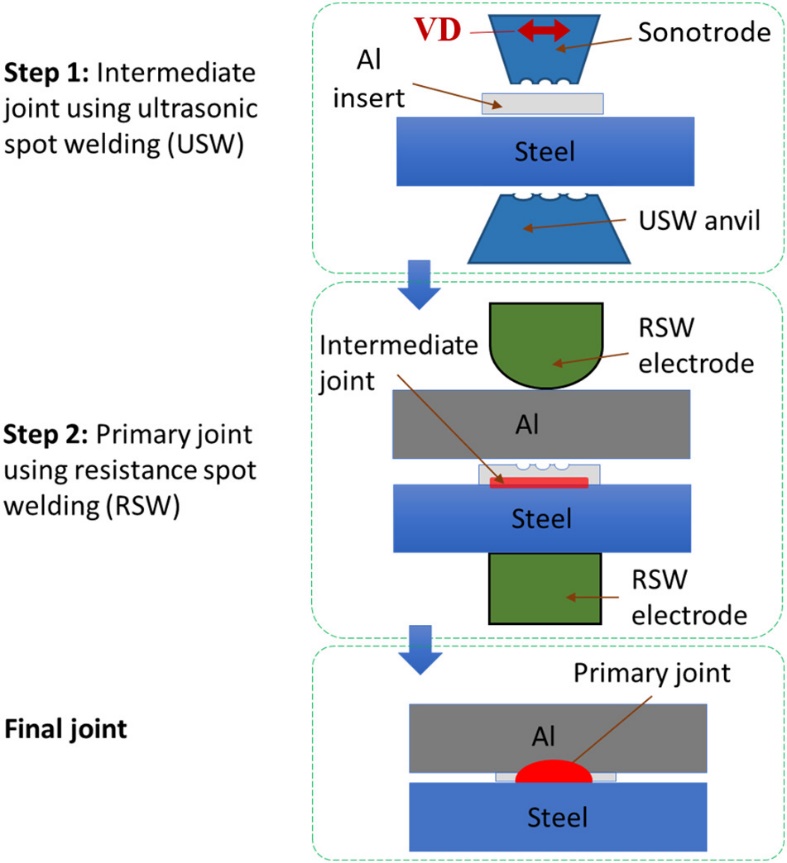
Figure 1: Schematic diagram of U + RSW process. VD is the sonotrode’s vibration direction for USW of intermediate joint.L-10
The U+RSW method showed improved mechanical properties (Figure 2), tensile shear strength and energy absorbed prior to failure. It has shown a relatively thin intermetallic layer.
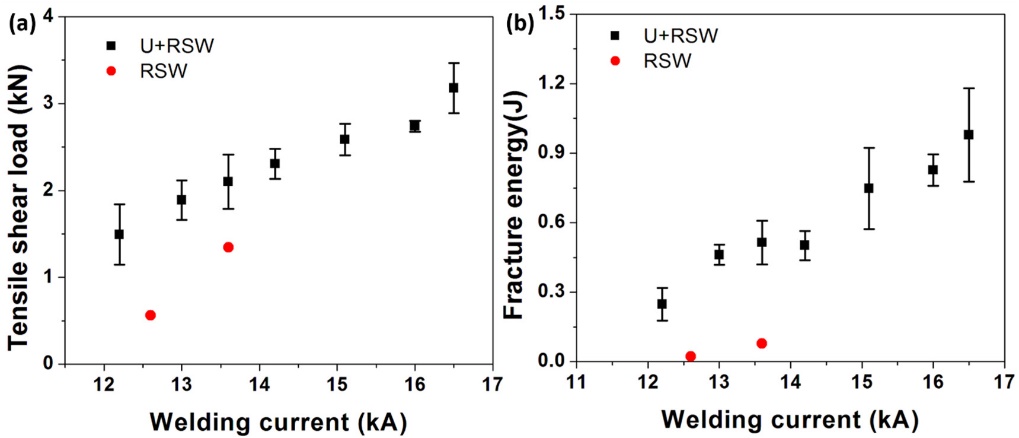
Figure 2: Effect of welding current on tensile shear strength and fracture energy of welded dissimilar joints of AA6061 to AISI 1008 steel. The welding time and the electrode force for RSW were kept constant at 0.083 s and 3.56 kN, respectively.L-10
Another research group have developed ultrasonic resistance welding process (URW), that apply in situ ultrasonic waves during the resistance spot welding process.S-17 The schematics of the process is shown in the Figure 3. Al-6061T6 and electrogalvanized TRIP 780 with dimensions of 70Lx 25Wx 1.6T mm3 and 70Lx 25Wx 1.3T mm3 respectively were used in this study.
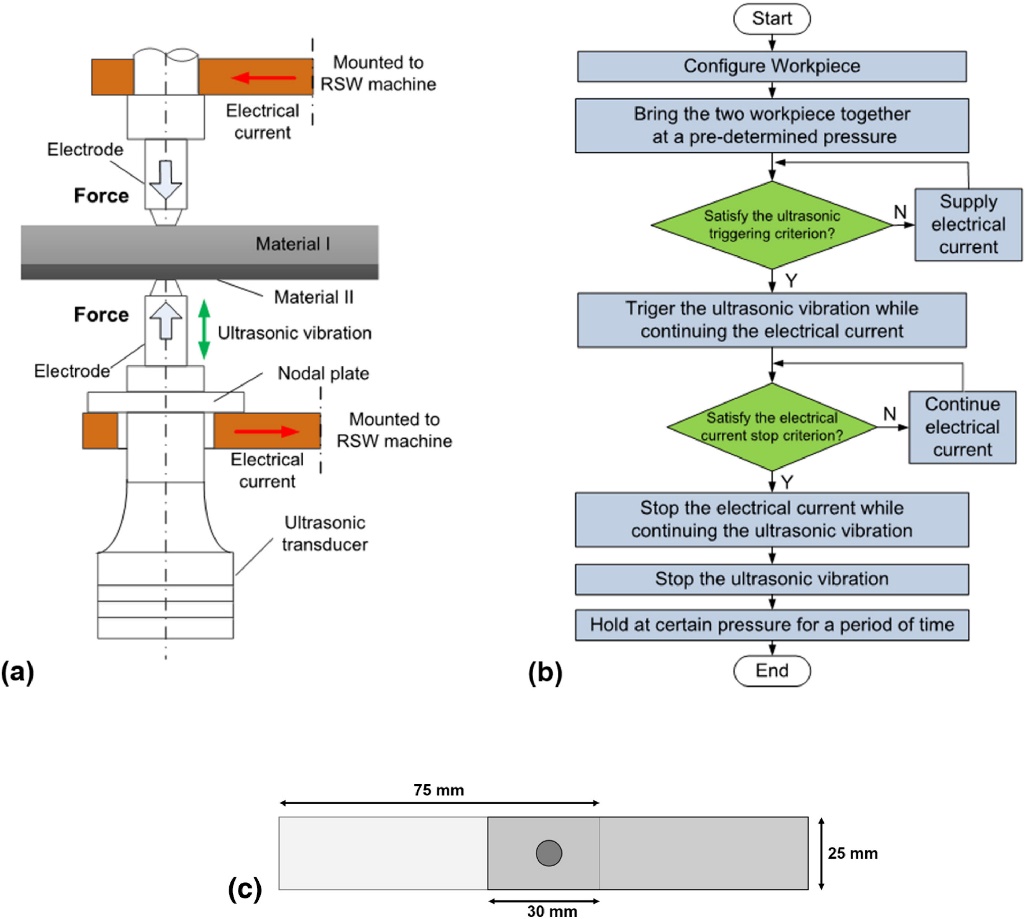
Figure 3: URW Setup (a) Schematic illustration; (b) Flowchart of the URW process.S-17
The URW process have shown improved mechanical properties and defect free interface compared to the RSW process. In situ ultrasonic vibration facilitated the breakdown of the surface oxide and contaminants, which modifies contact resistance and heat generation rate accordingly. If melting occurs, ultrasonic vibration promoted wetting of the molten aluminium over the steel interface and resulted in the formation of defect-free, fully bonded interface as shown in Figure 4. Figure 5 compares the mechanical properties between the RSW & URW process. Comparing URW with traditional RSW, up to 300% increase in strength and more than 150% increase in displacement to failure is reported. SEM images of the fractured surface revealed that ultrasonic waves eliminated the eggcrate morphology generally observed in the RSW welds fractured surface, which is a typical representative of solidification cracking.
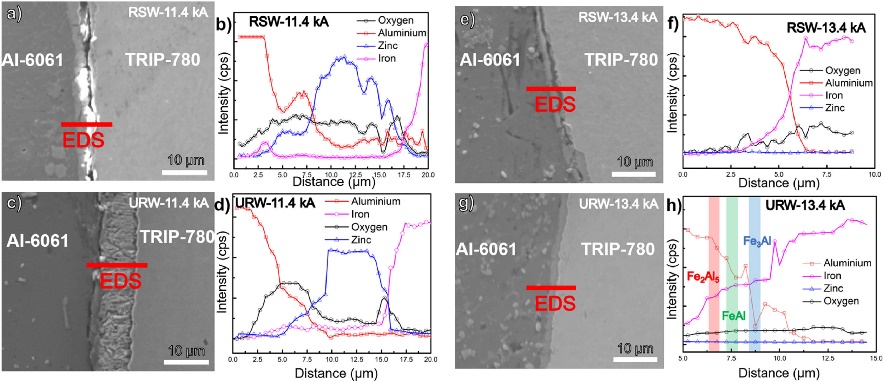
Figure 4: EDS line Scan of Al-6061 & TRIP 780 across interface for RSW at 11.4 kA (a-b) & at 13.4 kA (e-f) and for URW at 11.4 kA (c-d) & at 13.4 kA (g-h).S-17
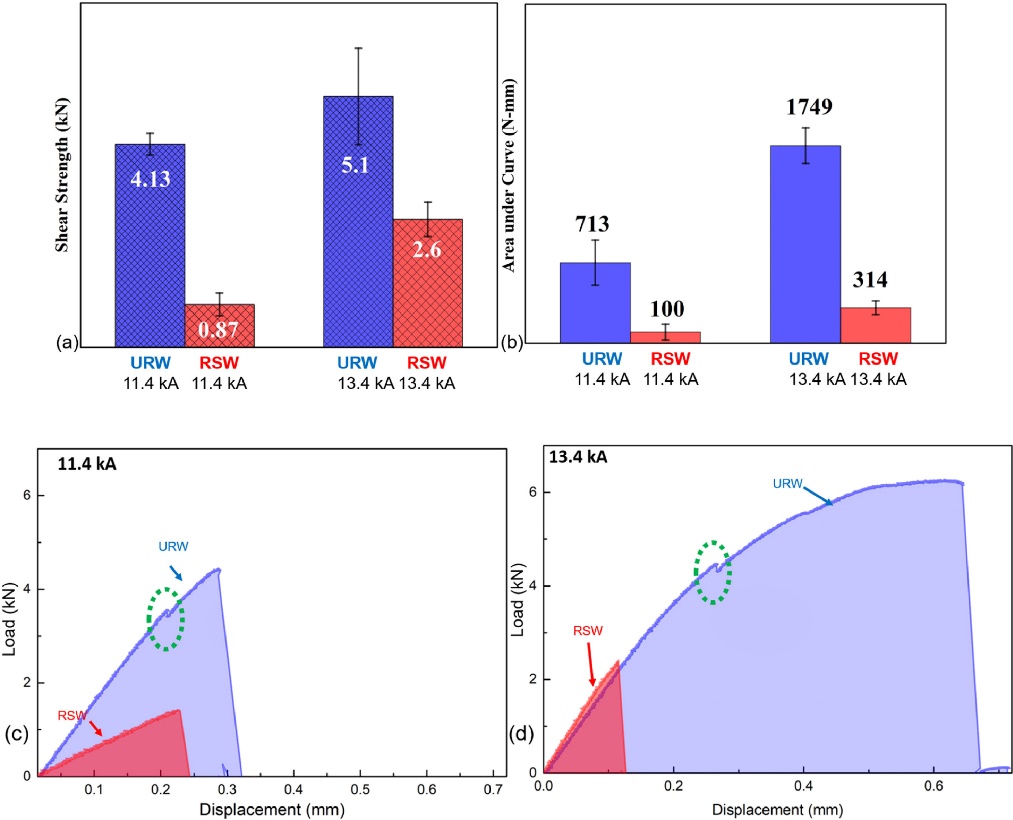
Figure 5: Tensile test results (a) Joint shear strength from 11.4 kA (30 cycles) & 13.4 kA (20 cycles); (b) Energy absorption calculated via area under load-displacement curve for 11.4 kA (30 cycles) & 13.4 kA (20 cycles); (c-d) load-displacement curve for 11.4.S-17
Joining Dissimilar Materials, Resistance Welding Steel to Aluminium
In order to improve the microstructure and mechanical properties of Aluminium/Steel resistance spot weld joints, Qiu et al.Q-6 tried a new welding method called resistance element welding (REM), i.e, resistance spot welding with a rivet. In this joining method, a hole was drilled in the overlap area of aluminium alloy sheet and a steel rivet was inserted into the hole. Then resistance spot welding was conducted on the rivet. The schematic of the setup was shown in Figure 1. A 4 µm-thick FeAl IMC layer formed at the rivet/Al interface, while FeAl3 formed at the Aluminium/Steel interface. Maximum tensile shear strength of 3.85 kN and pull-out failure mode can be obtained with a welding current of 21 kA, which was much higher than without a rivet (2.8 kN). Crack propagated along the Aluminium/Steel interface until it was arrested by the rivet. Then the crack propagated along the Al/rivet interface until failure.
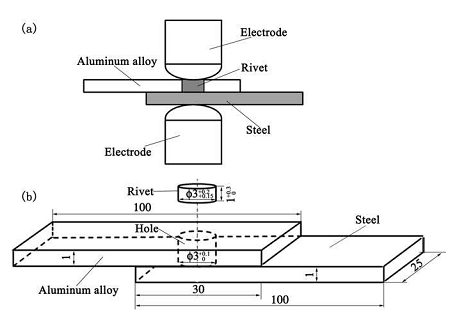
Figure 1: (a) Schematic diagram of resistance spot welding with a rivet; (b) configuration and dimension of the sample (in mm).L-9
Recently, Ling et al.L-9 tried resistance element welding of 2-mm-thick 6061-T6 aluminium alloy with a 1.4-mm-thick galvanized DP780 Dual Phase steel with a 5-mm diameter Q235 steel rivet. With optimum welding conditions, the tensile shear strength of resistance element welded Aluminium/Steel can be 7.368 KN with a fracture energy of 18.9 kN, which were 70% and over 6 times higher than those of RSW joints (Figure 2). The fatigue limit of REM joints was 1.8 kN, which was twice those of RSW joints.
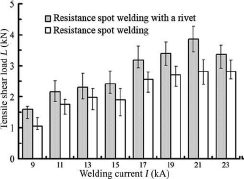
Figure 2: Effect of welding current on the tensile shear load of joints.L-9
Joining Dissimilar Materials, Resistance Welding Steel to Aluminium
In order to reduce welding current and improve heat balance during resistance spot welding of aluminium alloy to steel, Qiu et al.Q-1, Q-2, Q-3, Q-4, Q-5 has investigated resistance spot welding of Aluminium/Steel with a cover plate on the aluminium alloy side. A 1 mm A5052 sheet and 1 mm cold-rolled steel (SPCC) sheet were resistance spot welded with a steel cover plate. The schematic diagram of the process is shown in Figure 1. The IMC layer composed of a tongue-like morphology of Fe2Al5 on SPCC side and needle-like morphology of FeAl3 on A5052 side.Q-1 With welding current of 9 kA, thickness of IMCs is higher than 1.5 µm at the center of the nugget and the thickness of IMCs gradually decrease with distance from the center and become discontinuous at the peripheral region (Figure 2). Peak load of cross-tension strength can be 0.77 kN with the welding current of 12 kA. A crack propagated at the peripheral of A5052 and through the reaction layer. Thus IMCs deteriorate the cross-tension strength as the IMC is thicker than 1.5 µm.Q-2 The tensile strength of resistance spot welded Aluminium/Steel was influenced by the fraction of discontinuous IMCs layer, and a strong Aluminium/Steel spot weld can be obtained with increasing discontinuous reaction layer fraction.Q-4
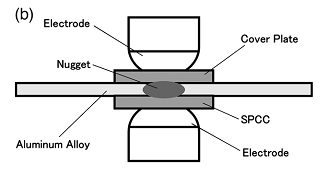
Figure 1: Schematic diagram showing resistance spot welding with a cover plate.Q-2
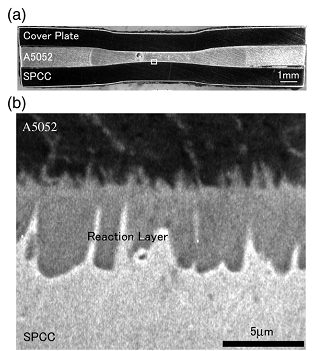
Figure 2: The cross-sectional macrostructure of spot weld joint (a) and SEM images of Al/steel interface.Q-2
Resistance spot welding of 1mm A5052 to 1mm SUS 304 with a cover plate was investigated and compared to RSW of A5052/SPCC.Q-3 At all welding currents, the IMC layer is thinner at the A5052/SUS304 interface than at the A5052/SPCC interface, since Cr in SUS304 can reduce the growth rate of Fe2Al5 (Figure 3). A maximum tensile shear strength can be 6.5 kN for A5052/SUS304 which is comparable to A5052/A5052, which is higher than that of A5052/SPCC (4.68 kN) (Figure 4). However, interfacial fraction occurs at Al/steel interfaces in both cases.
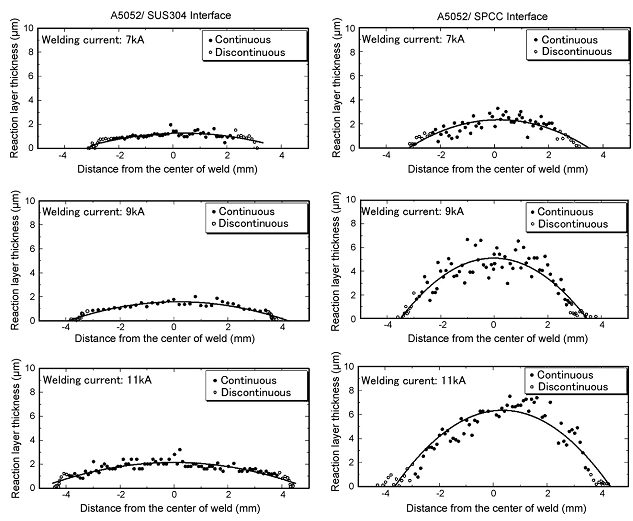
Figure 3: Distribution of intermetallic compound layer thickness at Al/steel spot weld interface.Q-3
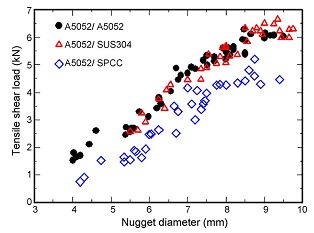
Figure 4: Relationship between nugget diameter and tensile shear strength.Q-3
Joining Dissimilar Materials, Resistance Welding Steel to Aluminium
In order to reduce the thickness of brittle intermetallic compound (IMC) formed at Aluminium/Steel interface to improve the mechanical properties of resistance spot welding of Aluminium/Steel, different interlayers, such as Al-cladded steelS-16, O-3, Y-4, Al-Si alloyW-15, Al-Mg alloyI-6, pure Al W-16, Zn coating.M-10, U-4, A-21 Sun et al.S-16 has done resistance spot welding of 1.4-mm SAE 1008 mild steel with 2-mm 5182-O aluminium alloy using 1 mm/1.5 mm cold rolled aluminium-clad steel as a transition metal with Aluminium/Steel thickness ratio of 20/80 (thickness ratio was not optimized). The formation of IMC cannot be observed at the interface for cold rolled aluminium-clad steel. The electrodes used were Class 2 30-deg truncated cone with 8-mm surface diameter and 3-inch face radius on the aluminium side and 30-deg truncated cone with 8-mm flat surface diameter on the steel side. Two separate nuggets created the aluminium and steel sides with a final nugget diameters of 10.1 mm and 8.2 mm, on aluminium and steel sides respectively, at the end of the 3rd pulse (Figure 1). With increasing welding time from 1 pulse to 3 pulses, the intermetallic compound layer thickness increased from 0 µm to 8.5 µm. As a comparison, the IMC layer thickness was approximately 65 µm for resistance spot welding of 1-mm AA 5052 and 0.8-mm low carbon steel without transition metal. The static and dynamic strength of the RSW samples in cross-tension and coach peel test were comparable to those of the peak load of self-piercing rivets (SPR). However, lap shear tensile testing of RSW samples had lower energy absorption than dissimilar SPR samples due to different failure modes (interfacial fracture may occur). Fatigue strength of RSW samples were lower than those of SPR samples due to stress concentration and tensile residual stress at the notch tip.
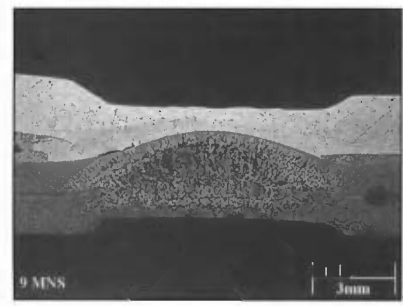
Figure 1: Cross-sectional macrostructure of resistance spot welding of SAE1008/A5182-O with 3 pulses of 12 cycles and welding current of 13.6 kA.S-16
Oikawa et al.O-3 have done resistance spot welding of 0.4 mm-thick cold rolled steel with 0.6 mm-thick pure aluminium with 0.77 mm-thick aluminium-clad steel sheet as the insert metal. Fe-Al IMCs have not been noted at the Aluminium/Steel interface of aluminium-clad steel sheet and a peel strength of 32.1 N/mm can be achieved. IMC layer thickness was about 5 µm at Al/steel interface when aluminium alloy and steel was resistance spot welded with aluminium clad steel sheet as insert. However, the tensile shear strength (Figure 2) and U-tension strength (Figure 3) can be 3.6 kN and 1.5 kN which were similar to Al/Al resistance spot weld joint and were much higher than direct resistance spot welding of 2.4 kN and 0.6 kN. However, it should be noted that resistance spot welding with aluminium-clad steel added weight, and difficulties in hot rolled aluminium cladding process result in high cost. Thus, more investigations have been done recently on improving the cladding method and using thinner and lighter metals as interlayers.
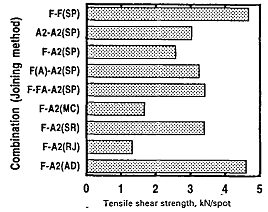
Figure 2: Comparison of tensile shear strength of Al/steel joints by various methods (SP-spot welding; MC-mechanical clinching; RJ-rivet joining; AD-adhesion bonding).O-3
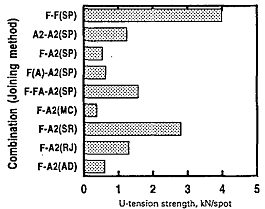
Figure 3: Comparison of U-tension strength of Al/steel joints by various methods (SP-spot welding; MC-mechanical clinching; RJ-rivet joining; AD-adhesion bonding).O-3
In order to reduce the IMC layer thickness, Zhang et al.W-15 used 4047 AlSi12 as an interlayer to suppress the IMC growth during resistance spot welding of 1 mm H22YD-Z100 Zn-coated high-strength steel sheets with 1.5 mm EN AW 6008-T66 aluminium alloy sheets. The effect of different interlayer thickness, i.e. 100 µm, 200 µm, 300 µm, 400 µm, on IMC growth behavior and mechanical properties were investigated. IMCs consisted of Fe2(Al,Si)5 and Fe4(Al,Si)13 and the thickness of IMCs reduced from 1.8 µm to 0.6 µm as the thickness of AlSi12 interlayer, increasing from 100 µm to 400 µm. But all were much thinner compared to 4 µm for spot welds without an interlayer. Nugget diameter reduced with increasing interlayer thickness and maximum tensile shear strength of 6.2 kN and pull-out failure mode were obtained with an interlayer thickness of 300 µm.
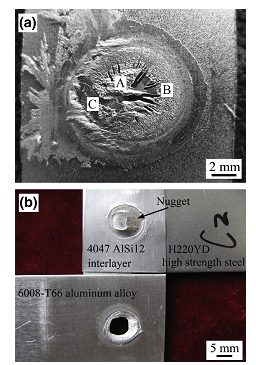
Figure 4: Fracture modes of H220YD high-strength steel/6008-T66 resistance spot weld joints (a) interfacial fracture without interlayer and (b) pull-out failure with 4047 AlSi12 interlayer thickness of 300 µm. W-15
Ibrahim et al.I-6 had investigated resistance spot welding of 2 mm A6061-T6 and 2 mm type 304 austenitic stainless steel with 80 µm thick Al-Mg alloy (80 wt % Al and 20 wt% Mg). Under the same welding condition, lap shear tensile strength was higher for welds with an Al-Mg interlayer than without the interlayer, and a peak strength of 8.4 kN can be obtained with welding current of 16.1 kA and welding force of 9.3 kN. A thin IMC layer of approximately 2 µm can be observed, which lead to a high strength. Fatigue strength of dissimilar RSW Aluminium/Steel samples were higher than Friction Stir Spot Welding samples. The failure mode depended on the load level. Pull-out failure occurred at high load (Pmax > 3 kN), shear fracture at 2.25 kN < Pmax <3 kN, upper Al sheet fracture at Pmax < 2.25 kN.
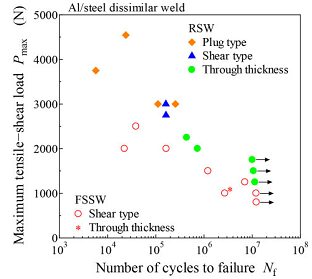
Figure 5: Relationship between peak load and number of cycles.I-6
Galvanized steel (GI) and Galvannealed steel (GA) are widely used in the automotive industry, and the presence of Zn layer may affect the weldability of Aluminium/Steel. Al-Zn eutectic liquid formed at Aluminium/Steel interface can efficiently remove oxide film and squeezed outside by electrode force. Thus, metallic surface of aluminium and steel can be revealed, and the Al-Fe IMC layer formed and a metallurgical bond was created.M-10, U-4 Arghavani et al.A-21 have investigated the resistance spot welding of 2 mm Al-5052 to 1 mm low carbon galvanized steel (ASTM A653 CS Type B-G60) (GI/Al) and compared that with the weldability of Al 5052 to uncoated steel DC01 (PS/Al). It showed the IMC layer at the GS/Al interface with the tongue-like layer of Fe2Al5 on the steel side and needle-like FeAl3 on the aluminium alloy side, as normally observed in Aluminium/Steel dissimilar welding (Figure 6).Z-3, U-4 GI/Al joints had thinner IMC layers at almost all the welding currents compared to PS/Al joints. And the IMC layer thickness in GI/Al increased initially with increasing welding current up to 12 kA and then decreased beyond it, which was due to heat consumption with Zn evaporation at higher welding current (I > 12 kA). Tensile shear strength of GI/Al welds were lower than PS/Al welds at welding current less than 12 kA since lower current led to incomplete nugget at GI/Al welds. However, tensile shear strength of GI/Al welds exceeded PS/Al welds at higher welding current since IMC layer thickness was over the critical value of approximately 5.5 µm for PS/Al joints at the higher welding current (Figure 7). Both GI/Al and PS/Al had an interfacial fracture mode with crack initiation at the peripheral regions of the weld and propagated through the IMCs layer/aluminium sheet.
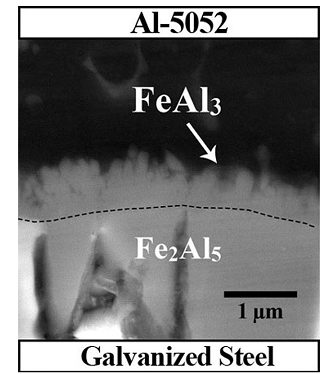
Figure 6: SEM image of IMC layer in GS/Al specimen.A-21
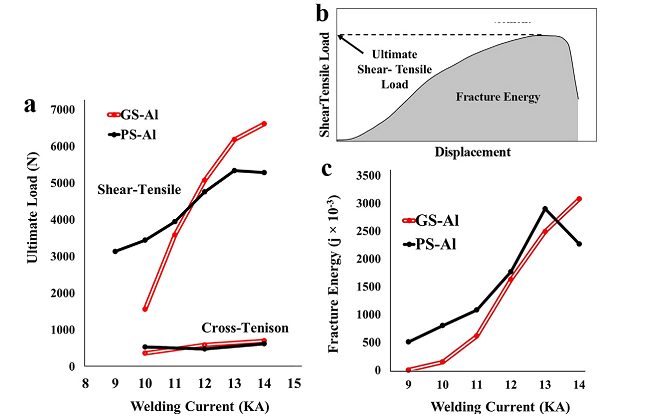
Figure 7: (a) Ultimate tensile shear and cross-tension strength vs welding current; (b) schematics of load-displacement curve and fracture energy calculation for tensile shear testing; (c) Fracture energy vs welding current.A-21
Joining Dissimilar Materials, Resistance Welding Steel to Aluminium
Capacitive Discharge Welding (CDW) is a similar process to RSW except large amount of energy is released in relatively a short amount of time. A research group at The Ohio State University working under supervision of Dr. Menachem Kimchi and Jerry Gould from EWI has investigated the feasibility of Al/Steel joining via this process. Figure 1 shows the current waveforms at 1.3ms & 2.5 and their mechanical strength results.
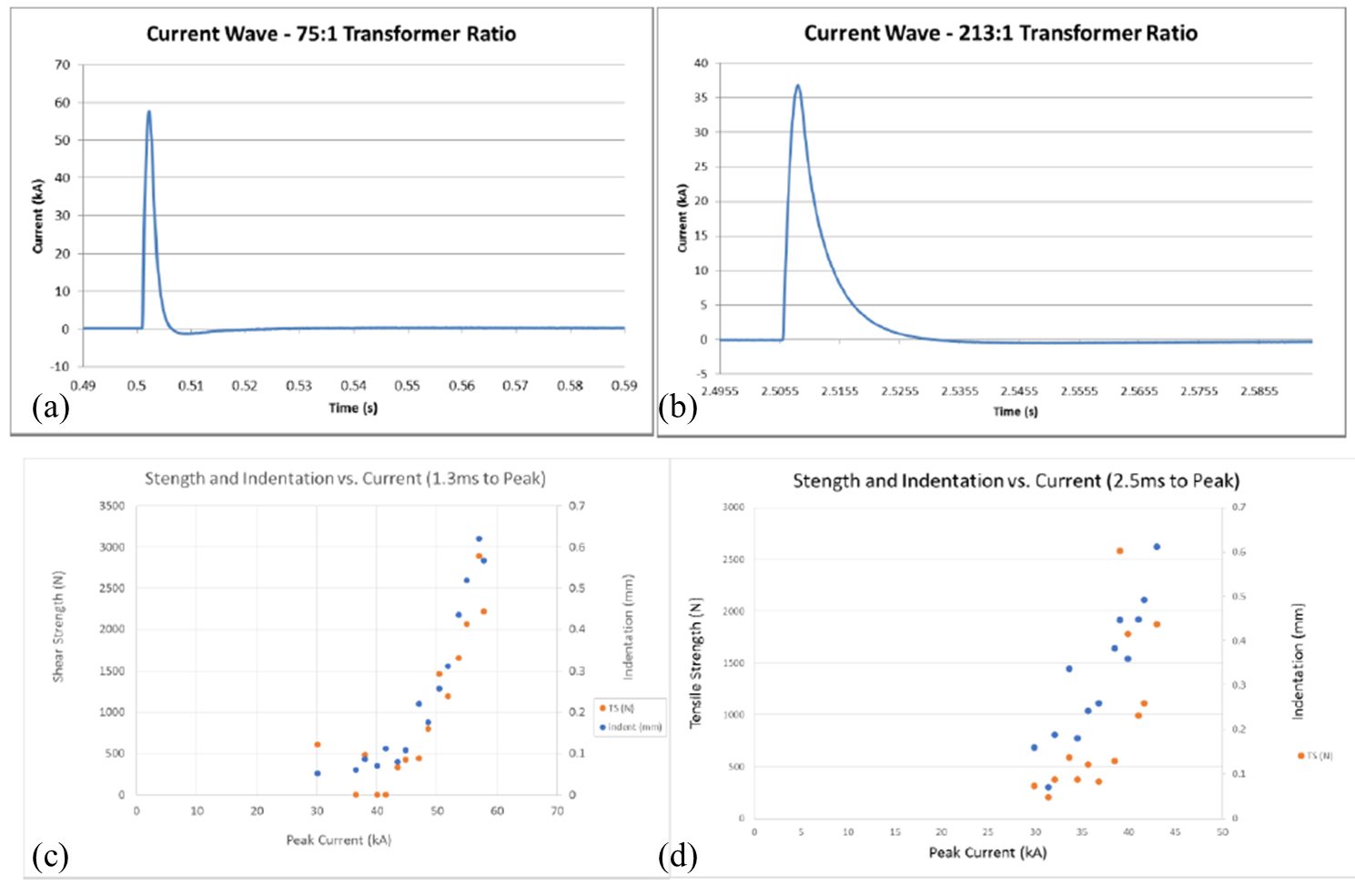
Figure 1: (a) Current waveform for 1.3ms to reach peak value, (b) Current waveform at for 2.5ms to reach peak value (c-d) Lap shear tensile strength and indentation as a function of current and time to reach peak current.
A shorter amount of time can significantly suppress the growth of the intermetallics. The group reported that higher amount of current in short duration of time could result in stronger welds. Figure 15 shows the effect of time on the growth of intermetallic (IMC) and solidification pattern. At 1.3 ms IMC layer thickness is less than 3 microns whereas at 2.5 ms the IMC layer thickness was reported greater than 3 microns. If IMC layer is less than 3 microns it results in nugget pull out failure mode whereas IMC layer of 3 microns or greater mostly result on interfacial failure.P-10
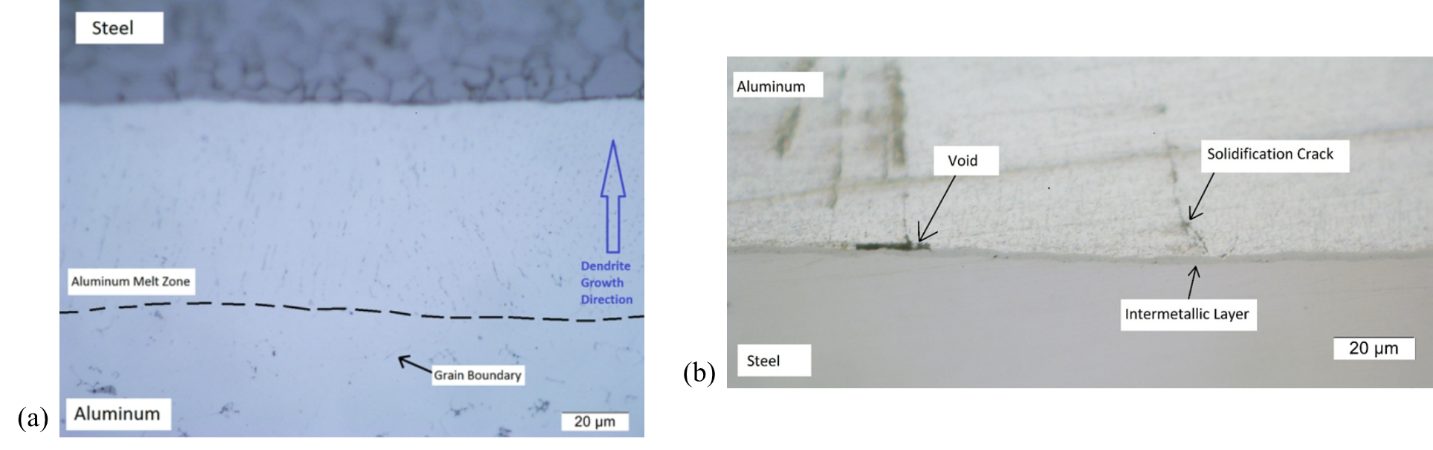
Figure 2: Effect of the ramp up time on the intermetallic layer thickness (a) 2.5ms & (b) 1.3ms.