Steel, and specifically advanced high strength steel, satisfies automotive industry requirements for safety, emissions, fuel efficiency, manufacturability, durability, and affordability.
Affordability
The automotive industry has adopted light-weighting as a key part of their greenhouse gas reduction strategy. This strategy, however, must be executed in an affordable manner.
Key reasons to deploy advanced high strength steels in automotive body structures include better performance in crash energy management and increased strength allowing this performance to be achieved with thinner materials, translating into lower vehicle weight. Thinner gauges mean a reduced amount of purchased material is needed to achieve this enhanced performance.
Today’s steels enable significant mass reduction, while meeting crash and other functional requirements, while preserving affordability. WorldAutoSteel vehicle programs and competitive benchmarking studies (here, here and here) have clearly demonstrated that optimized steel body structures can be constructed with little to no increases in total system cost relative to conventional body structures.
Recognizing these benefits, global automakers continue to base their fleet on steel-intensive body structures and rely on advanced high strength steel to help them meet customer expectations and government regulations in the most cost-effective way possible.
Most steel companies are extending their research and development efforts to expand the range of properties available through these new steels, to enable the production of safe and environmentally friendly vehicles.
Safety
The percentage of high strength steels used in light vehicles relative to other materials continues to grow, and much of the growth has been fueled by increasingly stringent safety regulations and ratings systems. Consumers are demanding safe cars, and governments are responding with new tests and standards that influence auto body structures, design and materials. The results of vehicle safety performance tests are therefore a strong incentive affecting consumer purchasing decisions.
In the United States, the National Highway Traffic Safety Administration (NHTSA) sets standards for vehicle safety, such as those for impact resistance, restraints, and fuel economy.[Citation 1] Testing by the U.S. Insurance Institute for Highway Safety (IIHS) has encouraged improved frontal, side, and rear impact ratings, as well as roof strength and rollover ratings, for vehicles on the road today.[Citation 2] Numerous New Car Assessment Programs around the world measures vehicle performance in a variety of crash tests, including front, side and pole impacts, and impacts with pedestrians with the goal of informing the public about the relative safety performance amongst vehicles. A limited number of these are shown in Citations 3, 4, and 5. Citations 6 and 7 summarize the testing that goes into these global New Car Assessment Program standards. Meeting these standards and improving performance often requires the addition of weight to the vehicle.
Diligent application of advanced steels leads to safe, lighter weight body structures. Tailoring the engineering design and forming approaches to the material characteristics and properties allows for optimized use of these newer grades and avoids excess or redundant mass in the structure. The FSV program showed that natural, non-linear load paths allow for more effective crash management and lighter weight structures, attainable through design optimization and rigorous application of AHSS.
Deploying Advanced Steels in Automotive Body Structures (3 subsections to follow)
Several key considerations drive material selection for automotive applications, including safety, fuel efficiency, environmental performance, manufacturability, durability, and quality. For exposed parts, aesthetic concerns related to paint finish and dent resistance are also important. These factors manifest themselves differently in each component of the vehicle, and materials are selected to match each set of performance requirements in the most efficient means possible.
Crash Performance
Perhaps the most critical design considerations for a vehicle structure relate to its ability to carry the required static and dynamic loads, particularly during a crash. Both materials strategy and geometric design play important roles in determining the final load paths and part details.
Two generalized areas of the car have very different safety requirements, as shown in Figure 1. The passenger compartment, enclosed in a rigid “safety cage,” is designed to protect the passengers in the event of a low or high-speed crash; the structure should prevent any deformation or intrusions that would compromise the integrity of the cage structure and impinge on the space around the passengers.
“Crumple zones,” located at the front and rear of the vehicle, are designed to absorb as much energy as possible in the event of a front or rear collision. By absorbing the energy over a distance, the crumple zone will cushion the impact and help preserve the structural integrity of the passenger compartment.
Table 1 presents general guidelines for materials selection in the crumple zone and the passenger compartment.
Table 1: Steel Grade Strategies For Targeted Safety Performance
Crash Zone |
Performance Requirements During Crash | Material properties to meet need | Evidence of Performance | Potential Steel Selection |
Energy Absorbing Crumple Zone | High energy absorption over a distance | High work hardening, strength, and ductility | Large area under the stress-strain curve | Dual Phase, Complex Phase, Transformation-Induced Plasticity3rd Generation Steels |
Deformation Resisting Safety Cage | No deformation or intrusion | High yield strength | Highest tensile strength | Martensite, Press Hardening Hot Formed, >980 MPa Dual Phase, >980 MPa Complex Phase |
Automotive components are designed so that together they form a structure that meets all crash, safety, and functionality requirements, including those enforced by regional regulatory bodies and those set internally by car companies. Steel grade characteristics and properties guides the selection for specific applications, as shown schematically in Figure 2.[Citation 8]
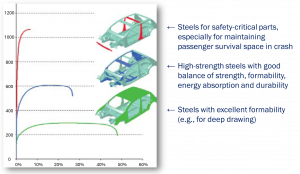
Figure 2: Comparison of stress-strain curves for different applications with body structures.[Citation 8]
Counter to the opinion of many, stiffness is not a function of a material’s strength. It is, however, a function of part geometry, elastic modulus and thickness. As an example of how part geometry influences stiffness, consider a sheet of paper. Stiffness improves dramatically once a crease is folded into it, yet the strength has not changed.
In automotive bodies, stiffness is related to handling and safety, as well as noise, vibration, and harshness characteristics.
A common strategy is to deploy AHSS for increased strength while decreasing weight by using thinner material. Casually applied, stiffness can suffer as a result. Geometry, specifically the moment of inertia of the cross-section about the primary load axis, plays a significant role in determining stiffness. The flexibility to adjust cross sectional and overall geometries allows for structural design solutions that more efficiently carry loads in the vehicle. The use of AHSS offers many advantages in this process because high work hardening rates increase formability, allowing for improved shapes for optimal efficiency. Additionally, AHSS typically possess high bake-hardening ability which can improve the final strength of a component after forming and paint-baking (curing).
Many lower-density metals like aluminium also have a lower elastic modulus than steels. To have an equally stiff component as one made of steel, the component from the lower-density material must have a combination of increased thickness or increased geometry. That increased thickness negates some of the perceived weight savings from the alternate material. Furthermore, lower-density materials must have sufficient ductility to be able to withstand the forming of the additional geometric features like in-part darts and beads and deeper draws associated with the increased shape required for stiffness improvement.
Forming and Manufacturability
Advanced high strength steels were developed partly to address the decreased formability typically associated with increased strength in conventional steels. AHSS grades are available at higher strength levels than conventional high strength steels, and have high work hardening and bake hardening characteristics that allow for increased formability and opportunities for additional optimization of part geometries. Both overall elongation and local elongation properties are important for formability, and global steelmakers have developed grades to address these needs.